Did the High Atlas really grow 20 cm in the Moroccan earthquake?
Well, almost - a small area did rise about 15 cm. Let's explore how we know.
Citation: Hubbard, J. and Bradley, K., 2023. Did the High Atlas really grow 20 cm in the Moroccan earthquake?. Earthquake Insights, https://doi.org/10.62481/9cb23fd9
We have made this post temporarily free so that everyone can learn about InSAR! Please consider becoming a paid subscriber to support free access to posts like this.
On Sunday, we received an email from one of our readers: Miloud Ait Slimane, a high school science teacher in Marrakesh. He wrote:
In recent days, I've come across some non-specialized media reports claiming, without geological analysis, that this earthquake may have caused a 20-centimeter increase in the height of the High Atlas Mountains. Are there scientific methods or approaches that can either confirm or refute this hypothesis? Furthermore, is it possible to assess the impact of the earthquake's intensity and rock mass movements on the shape and elevation of the terrain?
We love getting questions from readers - especially from people in regions affected by recent earthquakes.
So: did the High Atlas really grow by 20 cm? The short answer is: the mountains did get a bit higher in the rupture area - but by less than 20 cm - and this is a direct observation, not a hypothesis.
Why does the ground move up or down in an earthquake?
Earthquakes occur when a fault inside the Earth slips suddenly; the rocks on one side of the fault shift relative to those on the other side.
When that happens, the rocks around the fault warp. Some of that warping is up or down - which means that the Earth’s surface also moves up or down. This vertical movement is more intense in thrust or normal earthquakes compared to strike-slip earthquakes (where the movement is mostly horizontal).
For small earthquakes, the change in land surface might only be a few millimeters. In very large earthquakes, the land might go up or down by several meters! The depth of the earthquake is also important - very deep earthquakes may have only a tiny effect, while shallow ones can have more impact.
In some cases, when earthquakes occur below the sea, the movement of the ocean floor up or down can trigger tsunamis.
Even before there were any direct observations of uplift or subsidence in the Morocco earthquake, scientists had some guesses about the patterns we might see. Data from seismometers gave a “first guess” as to what kind of fault slipped to cause the earthquake, how much slip had occurred, and how deep it was. Using that information, scientists at the Istituto Nazionale di Geofisica e Vulcanologia (INGV) were able to model the expected movement of the Earth surface. They suggested that the maximum uplift would likely be ~13 cm.
The next thing to do was to compare this model to observations - but how?
How do we know how much the ground moves up or down?
Earthquake scientists use many different methods to measure changes in land height due to earthquakes. To give a sense of these, we will discuss three primary methods.
First, there are “natural” measurements, where we can see changes compared to fixed reference features like sea level. When areas near the ocean sink, the relative water level goes up - and areas that used to be dry are now below sea level. This is a great tool, because it can be used even in some ancient earthquakes, where trees died as they sank into salt water or where corals died after being lifted out of the water. However, these methods do not work for the recent earthquake in Morocco, which occurred in the high mountains.
Second, we can look at GPS measurements. If GPS stations are installed before the earthquake, the effect of the earthquake can be measured by using satellites to precisely locate each station. However, this works only in places where stations have been installed - not, as far as we know, in the High Atlas of Morocco. This also only gives point measurements, so many stations are needed to understand the full effect of an earthquake.
Third - and this is the source of the “20 cm” number - is Interferometric Synthetic Aperture Radar, or InSAR for short. This method uses images from a specific kind of satellite taken before and after the earthquake to look for changes in land height. Because the images can see a wide area of the land surface, this method can create maps of land height change, which is great!
How does InSAR work?
This method can seem really complicated, because the raw images look pretty weird. But it’s not so hard to understand.
InSAR images are created using two separate radar images taken at different times by a satellite. In each image, a radar wave is sent from the satellite toward the ground, and reflects back from a variety of points of Earth’s surface. The radar wave has a specific wavelength - the distance between two crests of the wave.
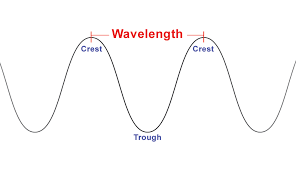
SAR satellites can generate signals with different wavelengths. For instance, the Sentinel-1 satellite has a C-band sensor with a wavelength of ~5.6 cm, and an L-band sensor with a wavelength of ~24 cm.
When the satellite captures an image of the land, each point on the land reflects the wave back with a different phase - a different stage of the wave, either at the trough, the crest, or somewhere in between. The sensor can detect this by measuring the amplitude of the wave - how strong it is. You could represent that reflected image as a map of phase (or amplitude), but it would not be very meaningful.
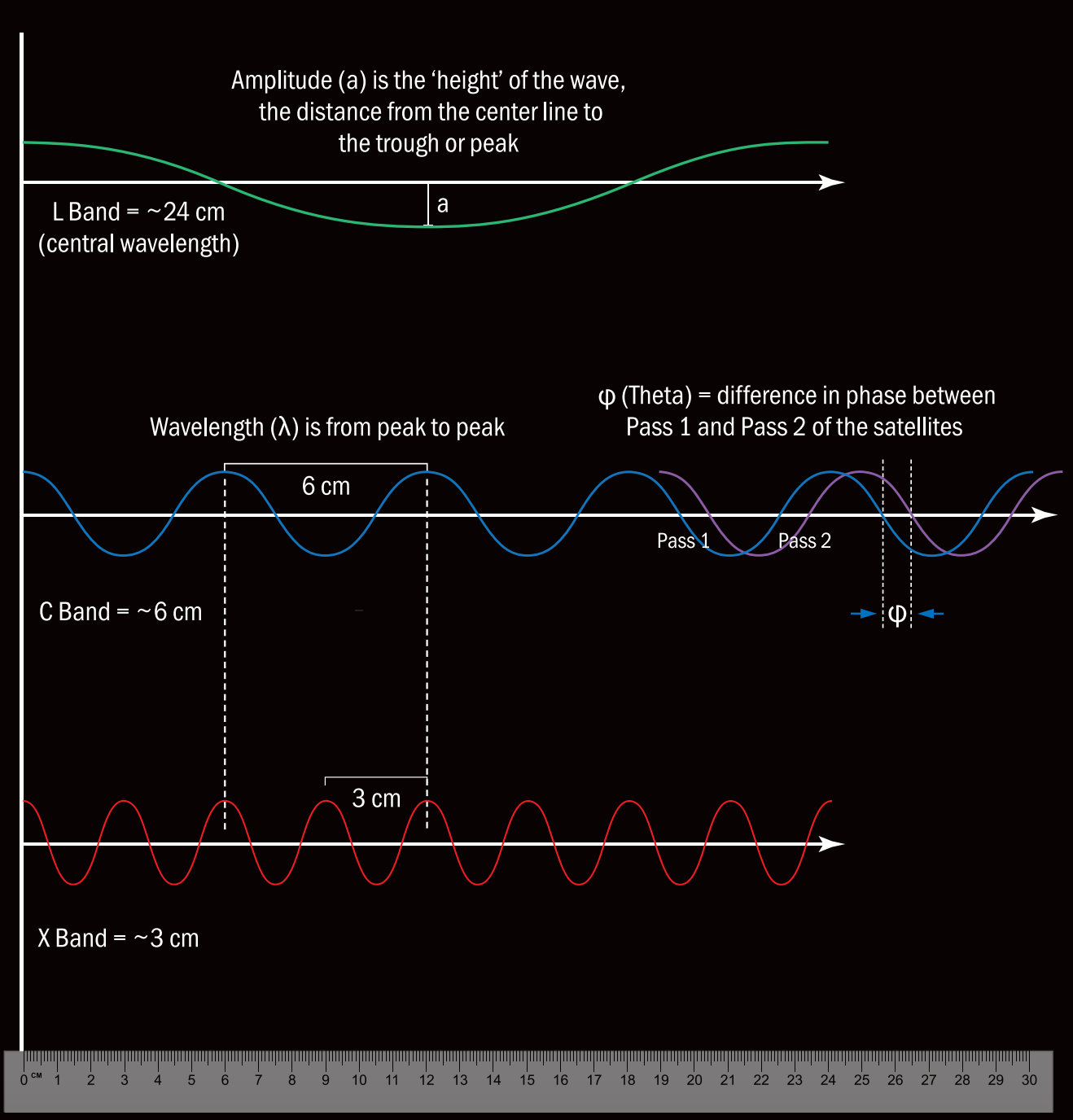
When the satellite passes over the same area of land some time later and takes another image, you can create a second map of phase. By comparing these two maps we are able to identify differences; this is a type of interferometry. If the land surface hasn’t changed, then this map should look just like the first one. If you subtracted them, you should get a map of zero - no phase change.
InSAR and earthquakes
But what if there has been an earthquake?
In that case, you should see some kind of change. Areas further from the earthquake might only show a little change - maybe the wave has only been shifted a tiny bit, and the total change is 1% of the wavelength. For the L-band sensor, 1% of the wavelength would mean a shift of ~2 mm.
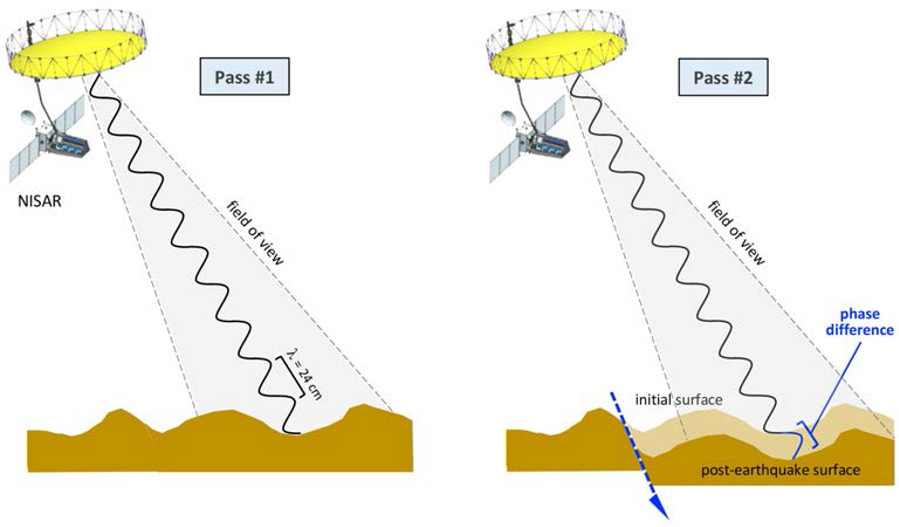
Areas that changed a lot, though, are a problem. If the change is more than half of the wavelength, the pattern starts to repeat - it’s not possible to tell the absolute change directly from the phase difference. (Half, because the sensors can’t tell the difference between positive and negative amplitudes.) If we make a map of the phase change, with different phases having different colors, we will see a pattern of colored bands called “fringes”. Each continuous color in a single fringe (say, the dark blue part of a fringe) represents the same amount of actual surface displacement.
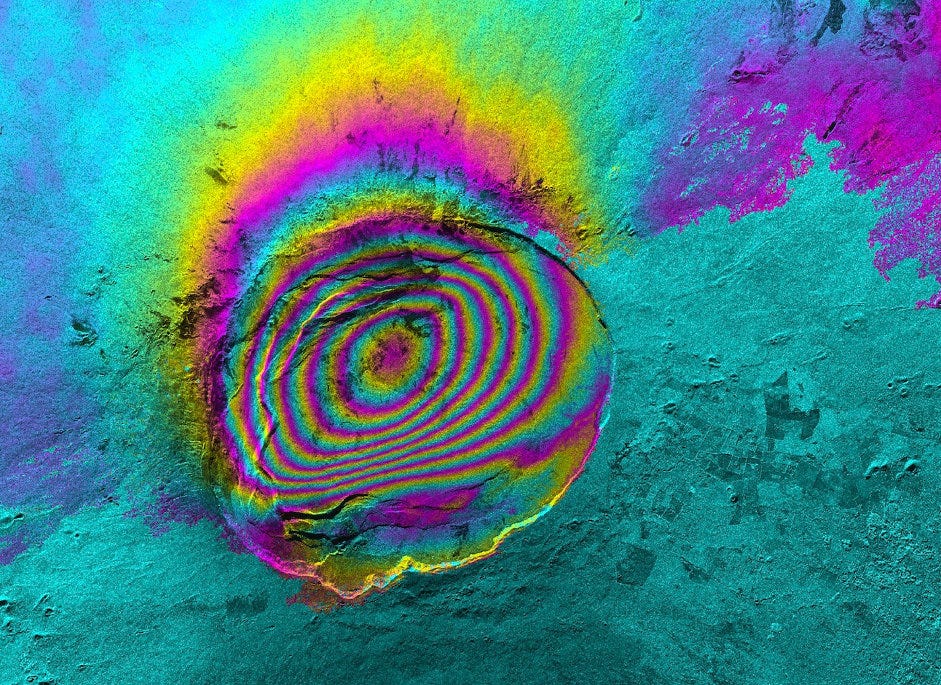
When an InSAR map is fringey, we can’t use point measurements directly - instead, we have to look at the whole map. The fringes are basically contours of change, and we can add them up to create a map of total change. This process is called unwrapping. Just like a birthday present!
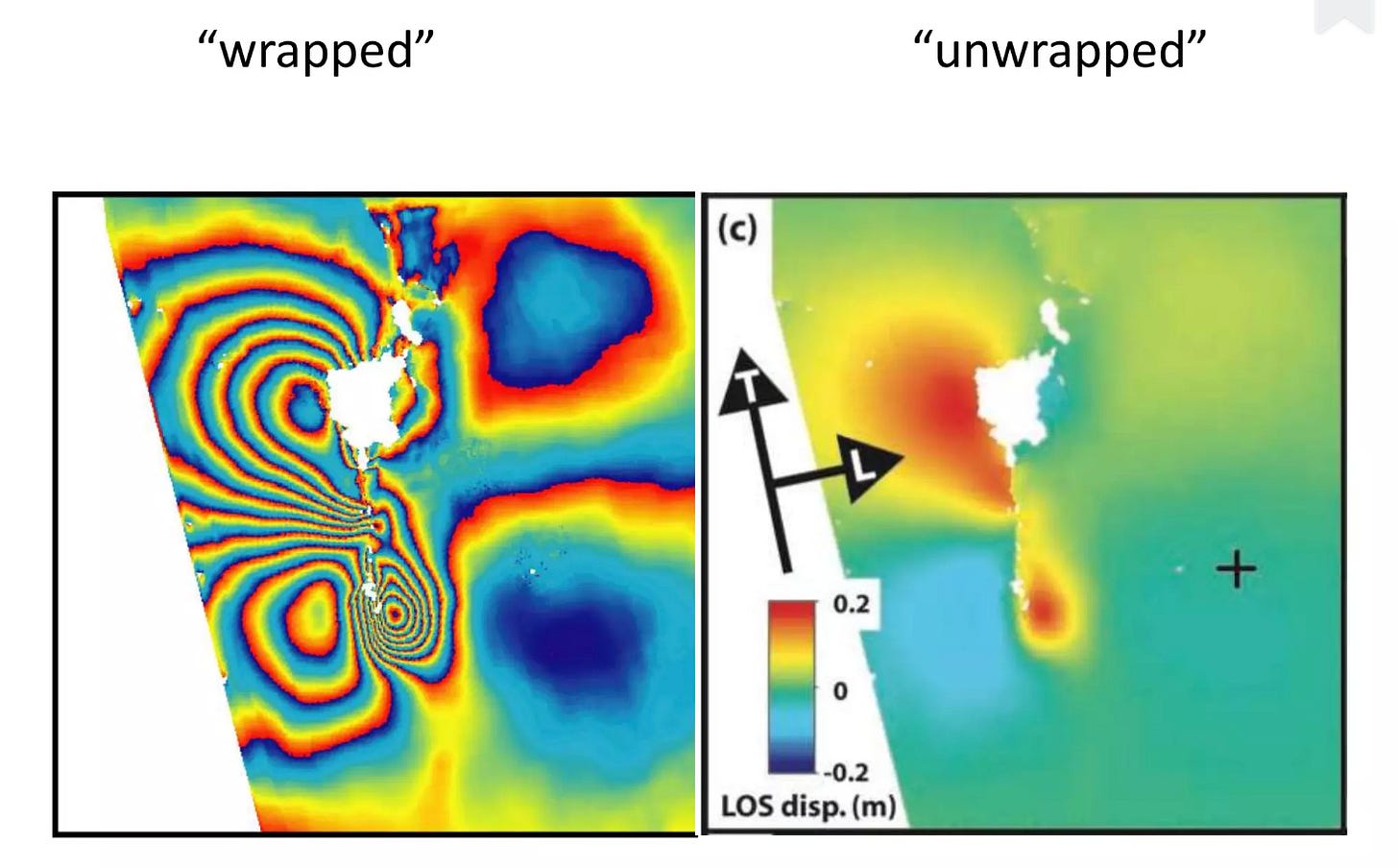
One cautionary note: InSAR satellites look down at the Earth’s surface from an angle - usually not straight down vertically. The changes measured by the satellite are therefore changes in the look direction. If the satellite is looking at a 45° angle towards the north, it is measuring changes in length along that line - not vertically. So, to calculate the try change in elevation, there have to be several measurements from different angles, which takes longer. This sounds complicated, but is actually quite smart. After all, if the satellite looked straight down, it wouldn’t be able to measure horizontal motions of flat areas very well.
So, what about Morocco?
The Sentinel-1A satellite passed over Morocco only a few days after the M6.8 earthquake, allowing the first InSAR images to be acquired. This isn’t by chance: these satellites are placed in specific orbits that allow them to monitor the whole Earth, but it takes a while for them to revisit a specific location. The images from Morocco looked like this:
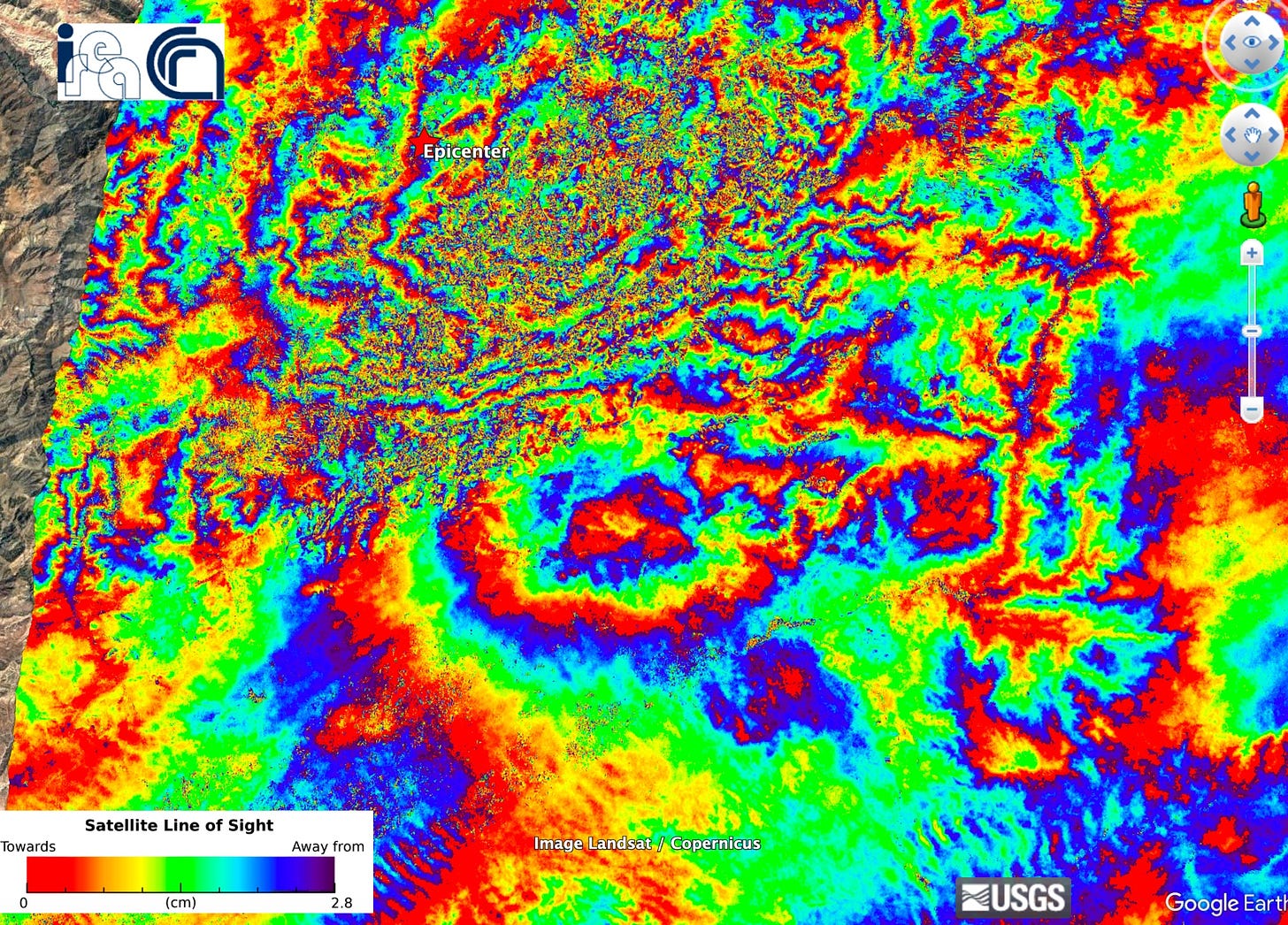
Messy! But fringes are still visible, if you squint. In the north, the fringes go red-yellow-green-blue away from the epicenter. In the south, the fringes go the opposite direction. This reflects the fact that areas above the epicenter went up, while areas to the southeast went down.
Why is the image so messy? It’s probably because of the topography. It’s much harder to get clean images in rugged terrain, like the High Atlas, than in flatter, smoother areas. In addition, rock falls and landslides cause problems. On top of that, there are effects from the atmosphere that need to be considered.
Still, researchers were able to unwrap the image.
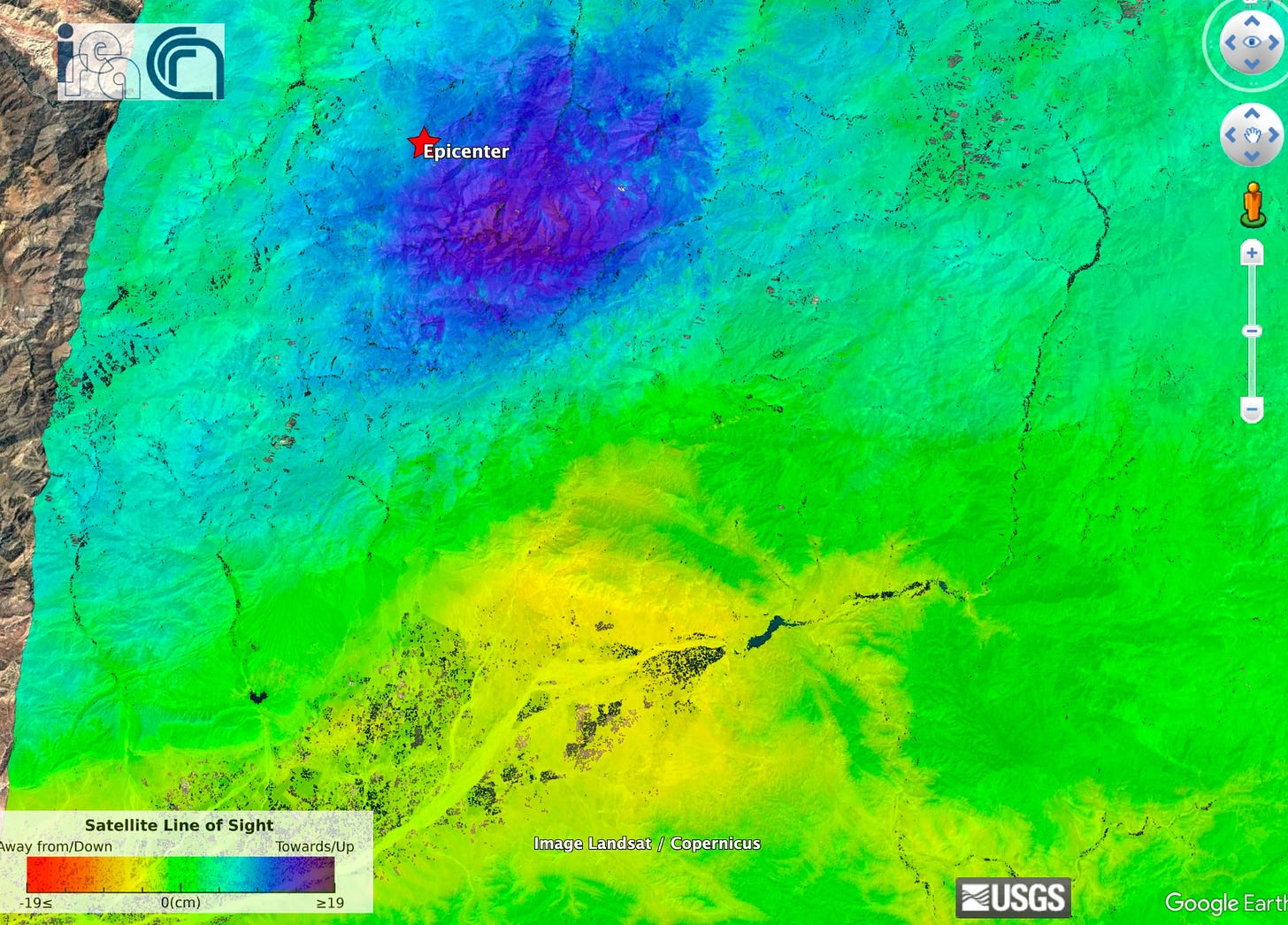
What does the unwrapped interferogram tell us? The purple areas - in a blob just southeast of the epicenter - represent ~19 cm of movement towards the satellite. This includes both the horizontal motion and the vertical motion.
Since most of the motion in this earthquake should be vertical - based on the model above - we can as a simple first step estimate how that motion towards the satellite might reflect uplift. The satellite looks down at the Earth’s surface at an angle of ~30-45°. For the interferogram above, the incidence angle is 39°.
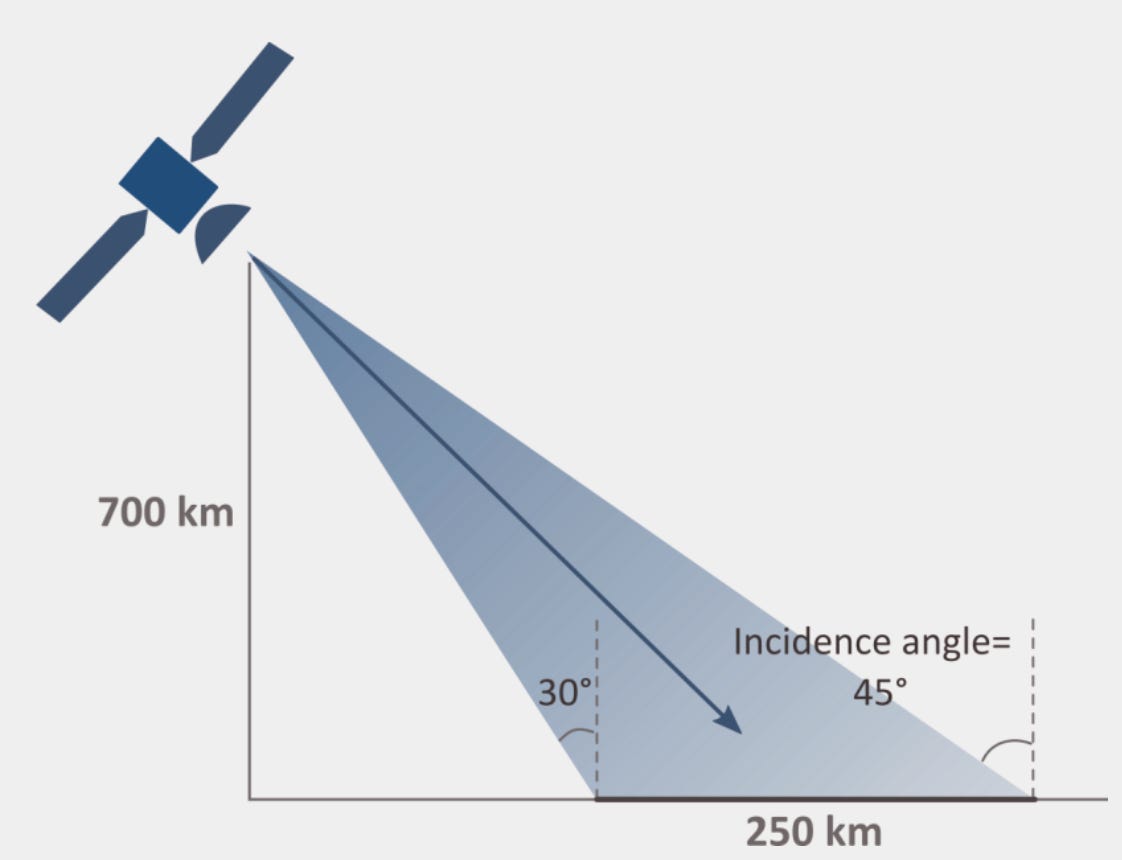
We can do some basic trigonometry - the vertical movement should be equal to the movement in the line-of-sight direction times the cosine of the incidence angle. So, an incidence angle of 39° and line-of-sight movement of 19 cm should represent ~15 cm of actual uplift. That is pretty close to the forward-modeled estimate based on seismic waves described above.
However, with only one image, it is actually mathematically impossible to separate the horizontal and vertical components. So measuring the true uplift requires more data - more images, taken at different angles.
Overall, we can say that the High Atlas grew ~15 cm, in a specific region near the earthquake. Some other areas at lower elevation sank ~4 cm. So far, we have not seen more precise calculations using multiple interferograms.
How can we use InSAR maps to understand earthquakes?
Even before satellite images were acquired above Morocco, researchers created forward models of what the InSAR maps might look like. A forward model uses some basic assumptions to generate a prediction.
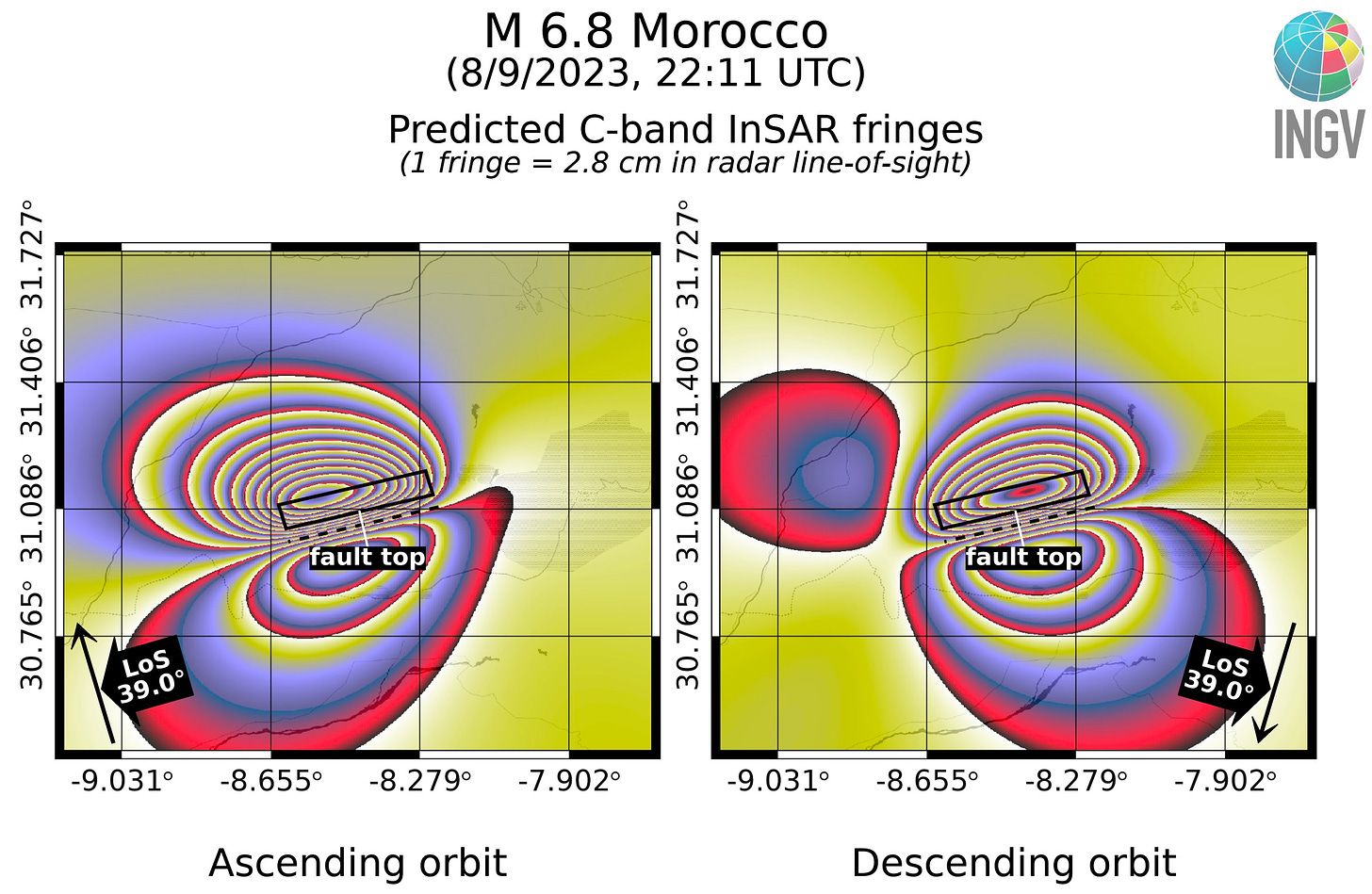
These models are based on the idea that we know generally how the crust flexes when there is slip on faults.
Earthquake scientists can work in the other direction, too. By looking at the deformation of the ground surface, they can infer what kind of slip must have happened at depth. This is called inversion - start with many data points and try to come up with the best fitting model that explains them.
This is the model of the earthquake slip developed by the Istituto Nazionale di Geofisica e Vulcanologia (INGV) based on InSAR imaging:
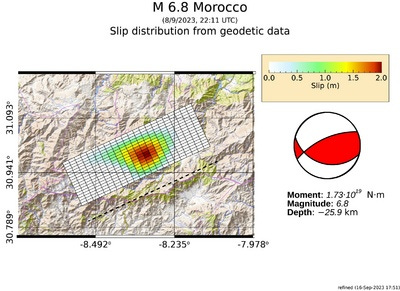
The fault seems to have slipped up to ~2 meters, with the hanging wall (northern side) moving up, and the footwall (southern side) moving down. That model is pretty close to the slip model calculated using seismic waves, which gives us confidence in the results.
Why was there only ~10-20 centimeters of upward movement at the surface, if there was ten times as much slip at depth? First, earthquake-related deformation is greatest near the fault; the movement of the rocks further away is progressively smaller and smaller with distance. Second, only some of the 2 meters was vertical movement; the rest was horizontal. Third, some of the slip involved the footwall moving down rather than the hanging wall moving up.
The growth of topography
Does that mean that over time and with additional earthquakes this specific part of the High Atlas will continue to grow, compared the rest of the range? No. Many things contribute to topography over geological time. Mountains typically reflect a balance between surface uplift (partially caused by earthquakes) and erosion.
First of all, we expect to see many different earthquakes occurring over geological time, on different parts of the fault and on different faults, each causing different changes in elevation.
Second, the areas further away from the faults are also moving, due to the slow movement of the tectonic plates. This incremental motion can be measured using GPS stations.
Third, high pmountains are usually supported by much deeper roots - just like icebergs, where the part above the water has to be gravitationally supported by a thick base below. So over many cycles, the apparent growth will be partially compensated by “settling” into the mantle. This process acts in reverse when a mountain range erodes.
Fourth, in the High Atlas in particular, there seems to be a dynamic upwelling of the mantle that is supporting the height of the range. The existence of this upwelling is partially inferred from the lack of a crustal root - a curious feature of this mountain range in particular!
Fifth, erosion keeps mountains in check by removing some of the material off the surface. So even a mountain range that is experiencing crustal uplift, may not exhibit a growth in elevation over long periods of time. However, erosion does not act equally on all rocks; some are stronger than others. Rainfall and glaciers also change the impact of erosion.
Disentangling all of these pieces is the work of tectonic geomorphology - the study of how plate tectonics and earthquakes change the shape of the land surface, and what the shape of the land surface therefore tells us about the past. The field must necessarily overlap with people studying seismology, mantle dynamics, remote sensing, hydrology, and more.
References:
Bradley, K. and Hubbard, J., 2023. Deadly M6.8 earthquake hits Morocco. Earthquake Insights, https://doi.org/10.62481/23bce686
Bradley, K. and Hubbard, J., 2023. Satellite images suggest slip on a steep, north-dipping fault in Morocco. Earthquake Insights, https://doi.org/10.62481/733c5fe2
Funning, G., 2018. How does InSAR work? SERC at Carleton College, https://www.slideshare.net/sercuser/presentation-on-the-background-theory-of-insar-96002109
Geological Survey of Norway, accessed 2024. What is InSAR? https://www.ngu.no/en/geologisk-kartlegging/what-insar
Teixell, A., Ayarza, P., Zeyen, H., Fernandez, M. and Arboleya, M.L., 2005. Effects of mantle upwelling in a compressional setting: the Atlas Mountains of Morocco. Terra Nova, 17(5), pp.456-461. https://doi.org/10.1111/j.1365-3121.2005.00633.x