One year after the February 6 Türkiye-Syria earthquakes
We look back at a year of aftershocks and initial scientific results
The experience of talking to - and hearing from - people impacted by the 2023 Türkiye-Syria earthquakes led directly to the creation of Earthquake Insights. We are grateful to those who have found value in our work, both here and via Twitter, and also to those from whom we have learned so much.
Bu yazıyı Türkçe (Google tarafından otomatik olarak tercüme edilmiştir) okumak için burayı tıklayın.
Citation: Hubbard, J. and Bradley, K., 2024. One year after the February 6 Türkiye-Syria earthquakes. Earthquake Insights, https://doi.org/10.62481/266f3dbf
One year has passed since two large fault ruptures occurred in southern Türkiye, both producing widely felt and damaging earthquakes. The magnitude 7.8 Pazarcık earthquake was followed nine hours later by the magnitude 7.5 Elbistan earthquake - now together known as the Kahramanmaraş earthquakes after one of the heavily damaged cities. The world was suddenly inundated with images and footage of destruction. The death toll rose to an eventual total of nearly 60,000 - a true disaster.
Images of the disaster zone, then and now, illustrate that even a full year after the earthquake, many areas remain fundamentally transformed. Hundreds of thousands of people remain displaced. As with most great earthquake events, the consequences will continue far longer than the aftershocks.
The earthquakes also ignited serious concerns among those who live along other large, active faults. People living in seismically hazardous places like Istanbul (on the North Anatolian Fault) and Beirut (on the Dead Sea Fault) are looking at the buildings around them with a renewed awareness of their vulnerability. We must choose to learn from disasters abroad, so that we won’t have to learn from personal tragedy.
Here, we will briefly review the seismic activity over the past year, in order to provide an updated picture of the ongoing earthquake sequence. We will then discuss some of the fascinating new observations and scientific ideas that are beginning to arise from the tremendous flood of information brought by these events.
A quick note - in the following maps, we plot hypocenters (circles) and focal mechanisms (beachball symbols) at their origin locations, which is the point on the fault where rupture first started. For very large events like the mainshocks, no single map symbol can accurately indicate the extent of the rupture, or the areas that experienced high ground shaking. As always, we welcome comments and suggestions for any improvements or necessary corrections. With that said, let’s look at some maps.
A year of aftershocks
Numerous aftershocks continue to illuminate both of the long and complex fault zones that ruptured. The most recent notable aftershock occurred less than two weeks ago: a magnitude 5.2 near Malatya, which caused strong shaking near its epicenter and was widely felt across the region. While a few large aftershocks within days to weeks of the mainshocks were damaging, most have been more minor. So far, more than 18,000 aftershocks have been recorded, although most have been far too small to feel. As more intense shaking has become less common, it has become possible to detect very small (M1) events. These were occurring before, too, but were too small to identify through the high background noise.
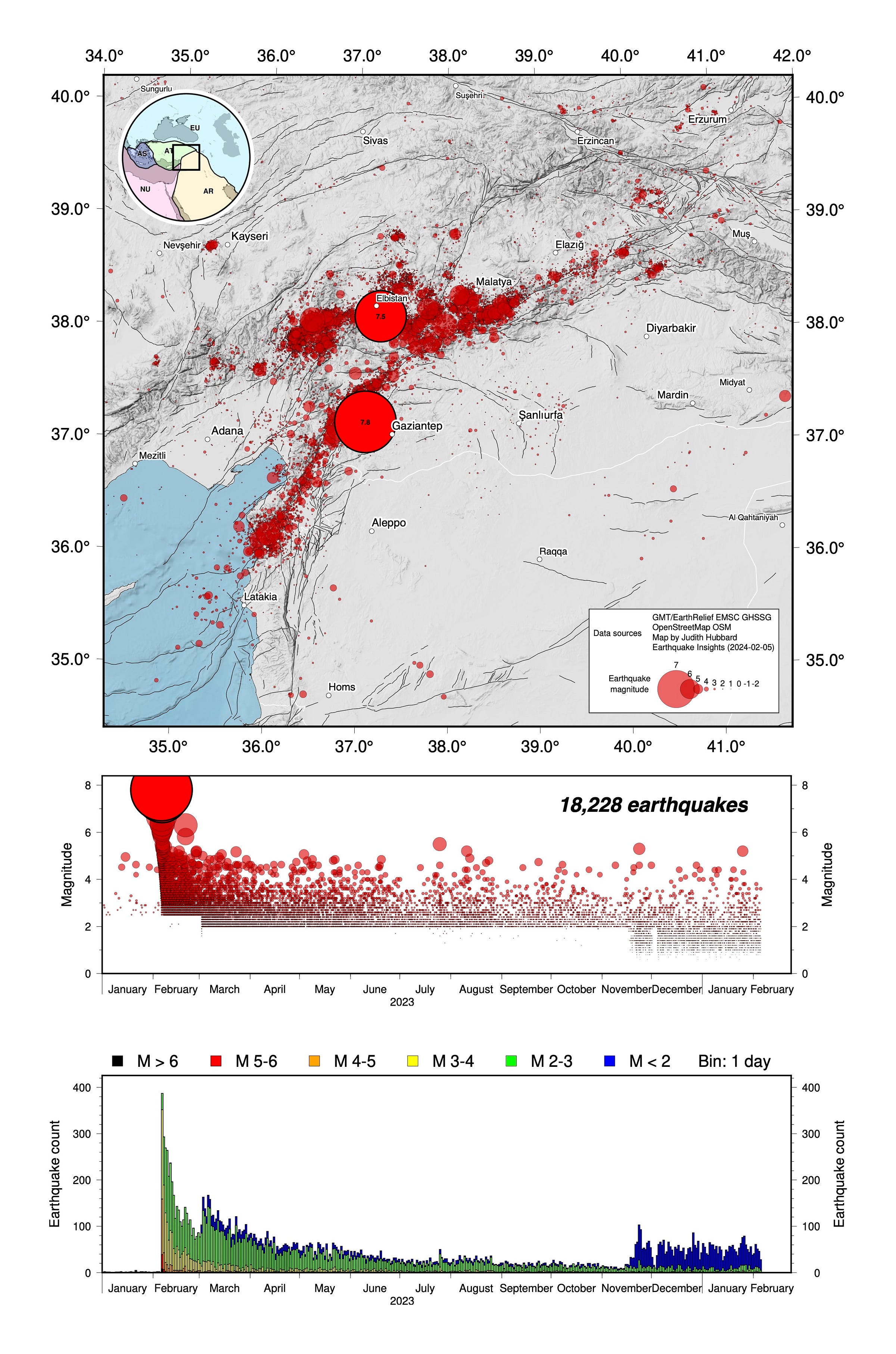
Most aftershocks will occur soon after mainshocks, with the subsequent rate of decay following a well known rule of thumb called Omori’s Law. In the following map, we have colored the earthquakes by time, with red events occurring most recently.
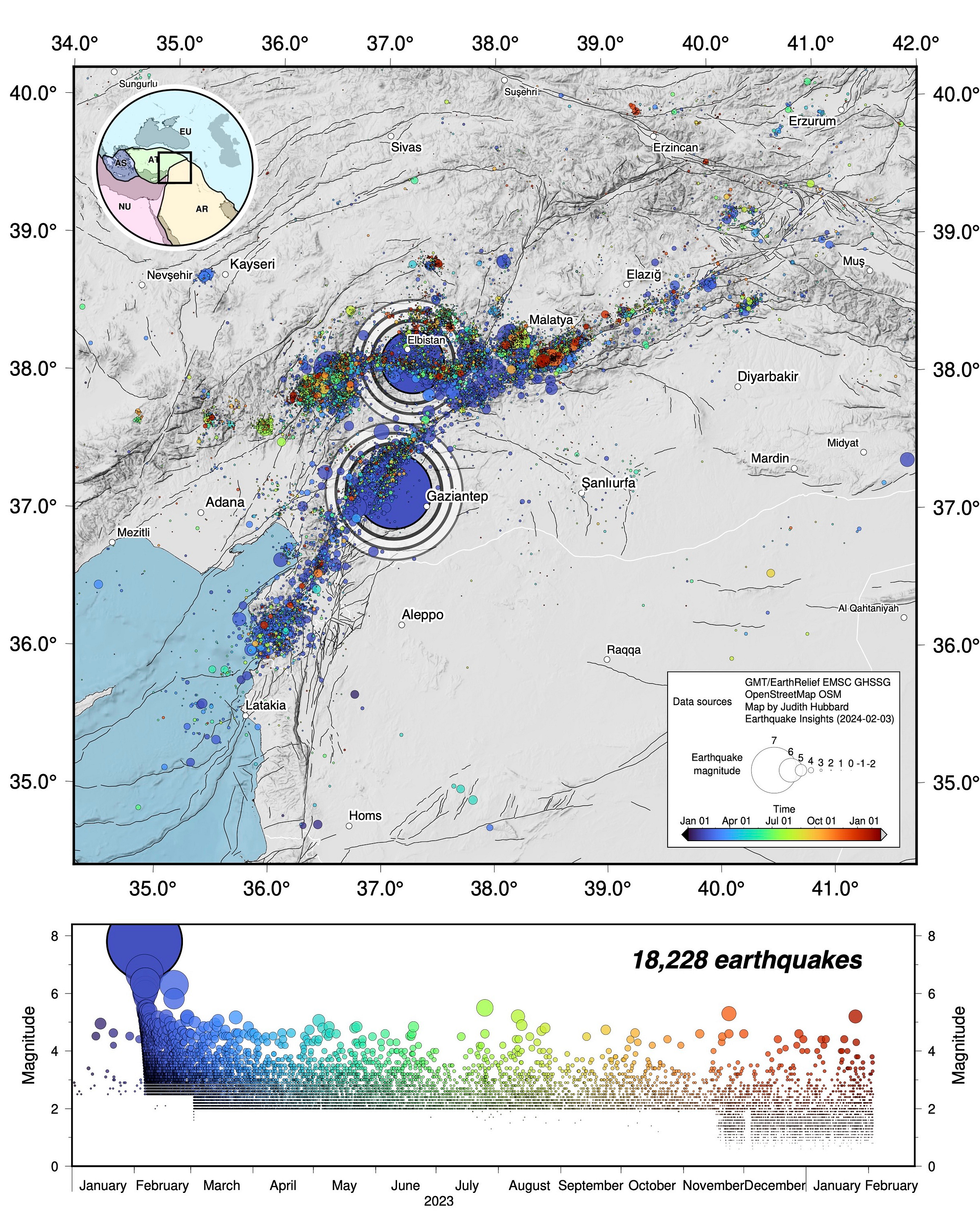
The more recent earthquakes are mostly concentrated near the tips of the ruptures. It is common to see high aftershock rates in these areas, because the Earth’s crust has been torqued but not broken by the nearby slip, causing many fault surfaces to approach their critical failure stress. Malatya, in particular, has had more than its share of aftershocks - being located at the tip of both the northeast-southwest Pazarcik earthquake, and the east-west Elbistan earthquake. In general, the fault that produced the Elbistan earthquake has been seismically noisier, perhaps because it experienced higher slip, concentrated on a smaller fault.
Let’s look at the aftershock patterns in more detail, on a region-by-region basis.
Northeastern region
This area has been the most seismically active in recent months, with the lastest event being a M5.2 southeast of Malatya. This region also includes the rupture area of the 2020 M6.8 Elaziğ earthquake - northeast of the more recent rupture - which here is notably silent. The lack of aftershocks along this part of the fault likely reflects the presence of a stress shadow: an area where the fault is under lower stress because of its previous rupture.
Northwestern region
This region includes the epicenter of the M7.5 Elbistan earthquake. The western edge of the rupture was notably active for months after the mainshocks, with many aftershocks occurring along NE-SW-trending normal faults. While these aftershocks have decayed in frequency over time, their slow westward movement is still visible as a small cluster of red-colored earthquakes in the far southwest corner. Fortunately, there hasn’t been an earthquake above M5 in this region for many months.
Central region
This region includes the epicenter of the M7.8 Pazarcık earthquake. After the first few days, the main fault in this area has been notably quiet. This may be due to a far-field stress-reduction effect by the subsequent Elbistan earthquake (see the following section on scientific results). The Pazarcık earthquake actually initiated on a smaller, secondary fault and then ruptured northward, where it triggered the main rupture on the East Anatolian Fault Zone. A M5.5 strike-slip earthquake on July 25 is included on this map, but occurred in the northwesternmost corner - a map of the area around Adana is included later.
Southwestern region
This region includes the February 22 M6.3 aftershock, which caused significant damage in Antakya and surrounding towns. Notably, many structures that survived the two mainshocks subsequently collapsed due to this much smaller magnitude, but closer aftershock. However, aftershocks have been well below M5 for many months. Seismicity offshore along the Cyprus Arc in the weeks after the mainshocks raised some concerns about the potential for an offshore triggered rupture - possibly tsunamigenic - but that activity has since died down.
Western region
Residents of Adana felt strong shaking during the mainshocks and largest aftershocks, but have thus far been spared a large nearby event. The M5.5 aftershock on July 25 is the largest earthquake to have occurred nearby during this sequence. Larger earthquakes in the vicinity of Adana remain possible - as demonstrated by a damaging M6.3 event in 1998.
A survey of some early scientific results
Large earthquakes spur scientific research for several reasons. The high degree of human impact obviously demands attention. Large events also produce a wealth of new data, allowing scientists to test long-standing hypotheses, improve models, and apply new methods. Finally, and perhaps most importantly, large earthquakes almost always challenge us with new fundamental questions about how the Earth works.
This earthquake sequence is being widely studied by the scientific community. The International Seismological Centre compiles a bibliography of papers for each large earthquake; their collection for the Kahramanmaraş earthquakes contains over 150 research papers, exploring aspects of geology, seismology, remote sensing, and engineering. A search on Google Scholar returns over 4,000 results - expanding the topic to include studies of post-traumatic stress, medical triage, humanitarian response, and tax revenues. The number of studies will undoubtedly multiply in years to come. So, what kind of earthquake science results are we starting to see?
The rupture process
Diego Melgar and colleagues published one of the first papers about the earthquakes - only five weeks after they happened. In their paper, they document the seismic sequence, and construct a model showing how each of the ruptures started and propagated along the faults. The first earthquake did not start on the East Anatolian Fault itself, but on a smaller splay to the south. When the rupture approached the main fault, it split, propagating in both directions. The second earthquake also ruptured in two directions, but at different velocities, moving much faster to the west than to the east. Slip on the faults reached up to 8 meters. These results have been improved on and refined by subsequent studies, but the basic story has remained the same.
The movie below shows the evoluton of the rupture. It is extremely useful because it illustrates how rupture of one specific section of a fault happens very quickly - the rupture front moves along the fault surface at a speed of several kilometers per second. The fault in one location may slide for only several seconds as the Earth adjusts, and sometimes the same section of fault may slip several times in pulses. The rupture of the entire fault takes around a minute, radiating new seismic waves as it advances. People experiencing the earthquake feel ground shaking for longer than the rupture takes, sometimes several minutes, as the surface waves interfere and reverberate.
Detailed rupture dynamics
A more recent study by Chunmei Ren and colleagues further explores the rupture dynamics, mapping out how rupture velocity changed along the fault. They identify a segment of the East Anatolian Fault, just east of where the originating splay intersects it, where the rupture reached supershear velocities. In other words, the rupture front outpaced the S-waves that it generated. This creates a type of underground “sonic boom” that has a variety of effects. Ren et al. indicate that this supershear rupture likely increased the intensity of shaking in the vicinity of the fault.
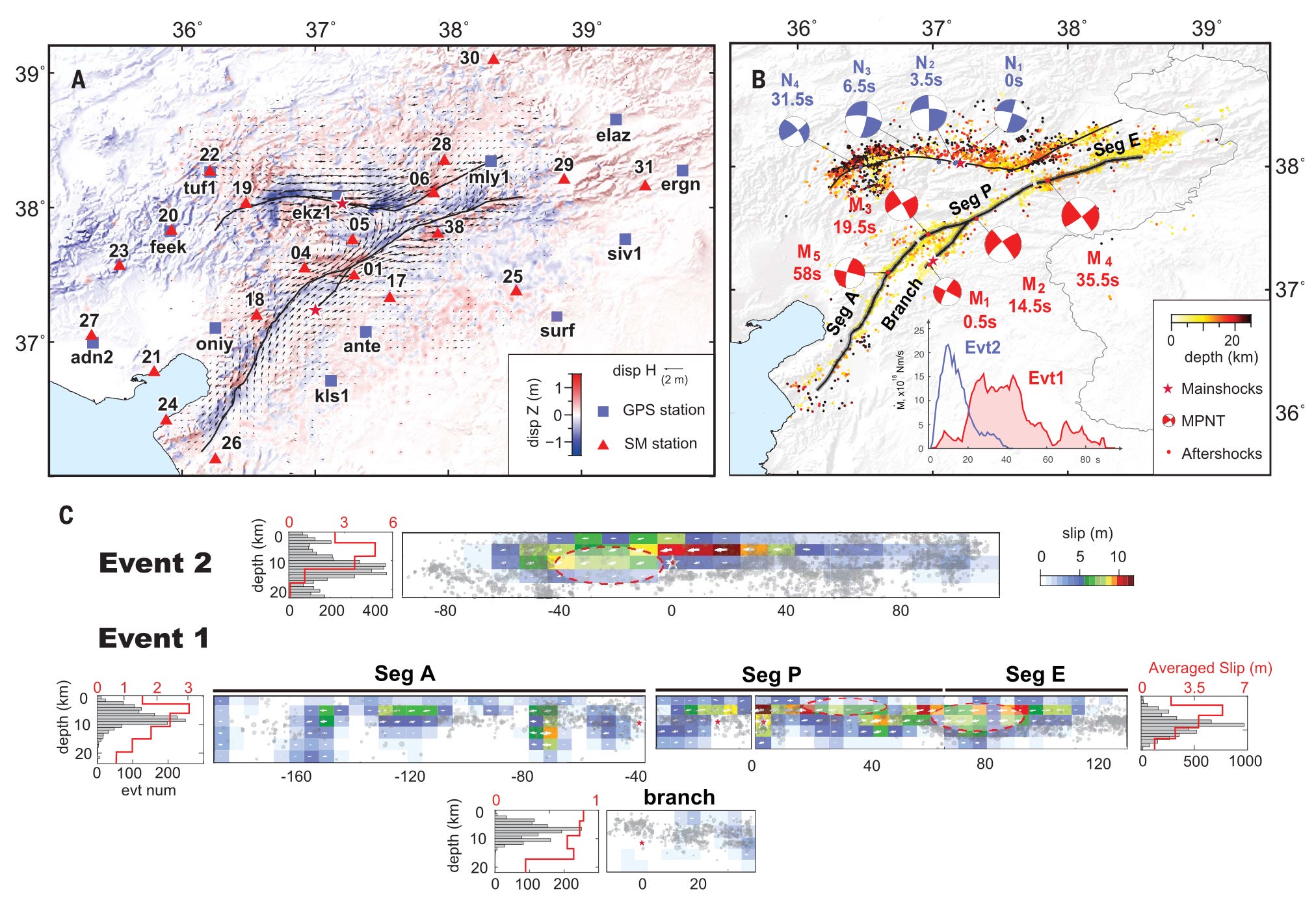
Intense shaking also linked to rupture stopping
P. Martin Mai and colleagues also explored the effects of how the rupture propagated, linking it to the intensity of shaking. They noted that shaking recorded by seismometers exceeded the expected value in some areas, sometimes by a significant margin. Based on their analysis, this effect was focused on the Hatay region - which did experience a huge amount of damage - because of how quickly the rupture decelerated in this region.
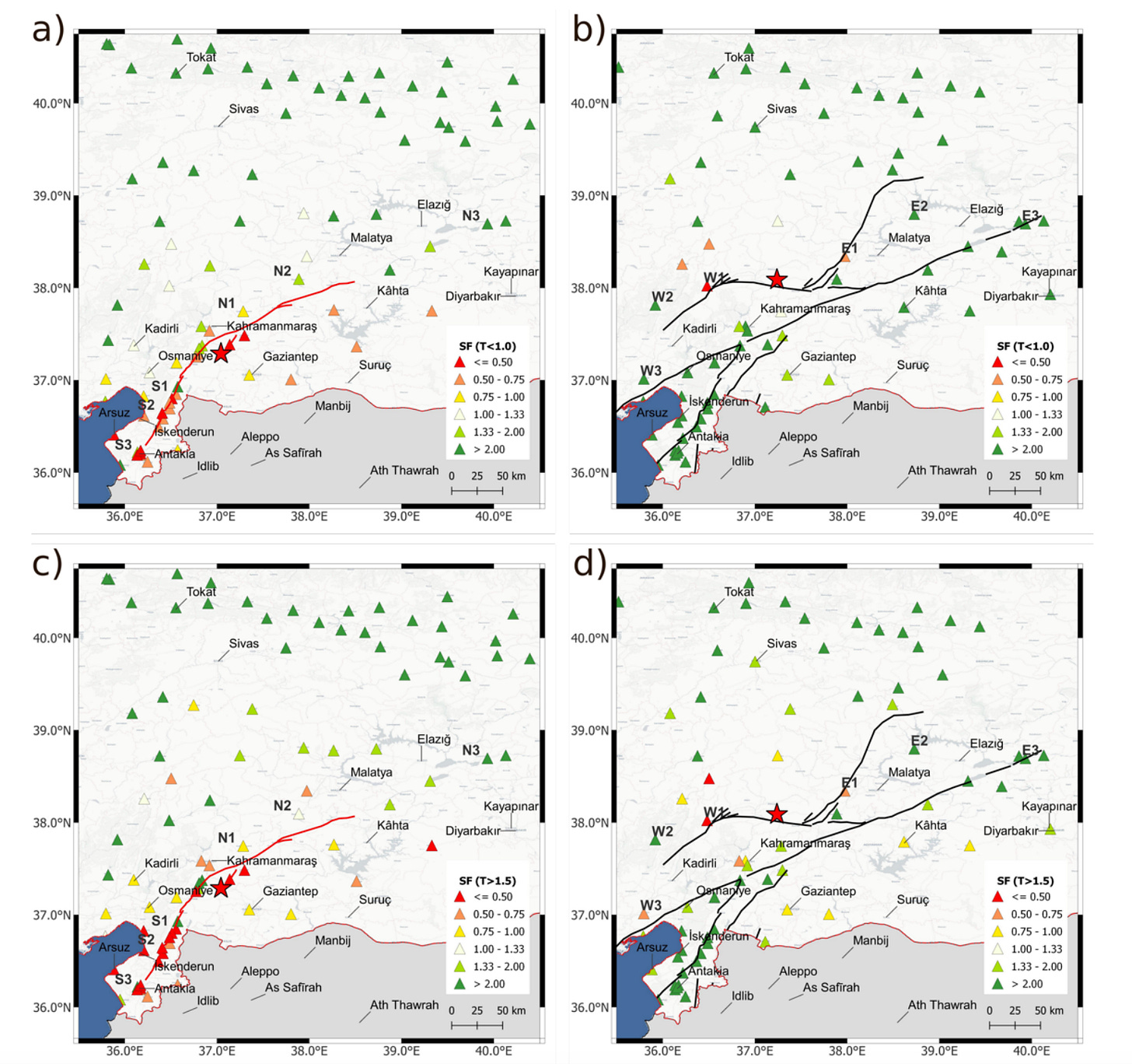
Earthquake interactions and stress changes
A paper by Shinji Toda and Ross Stein explores how earthquakes since 2020 have changed the stresses on faults in the area - making them more or less likely to slip. Based on their calculations, the M7.8 Pazarcık earthquake unclamped the part of the fault where the M7.5 Elbistan earthquake started, nine hours later.
There is a simple way of demonstrating the effectiveness of “unclamping” of faults. Have a friend press their hands tightly together in front of their chest, and then tell them to add some shear stress by trying to force one hand up and the other downward - but not enough to slide their hands apart. They are now a simulated fault. You can cause an earthquake by increasing the shear stress - you simply push one arm up and the other arm down until their hands slip apart - but it will be difficult if they are pushing their hands together hard. An easier way to break the contact is to quickly pull their wrists apart, toward their elbows. This reduces the "clamping force” - the normal stress - allowing the pre-existing shear stress to overcome friction and drive slip.
The story continues - the slip in the Elbistan earthquake then reduced the shear stress on the central Pazarcık segment, which had already ruptured; this explains why that part of the fault has produced so few aftershocks. Toda and Stein also link the low seismicity rate northeast of the ruptures to the 2020 M6.8 Elaziğ earthquake, which locally reduced the fault stresses. In general, they find that areas where the stresses make slip less likely are indeed beginning to produce fewer aftershocks.
Understanding why earthquakes occurred the way they did in the past also makes it possible to forecast the future - and that is what the authors do. The 12-month forecast of Toda and Stein (2023) started on December 1, 2023, and indicates that 1-3 M5+ earthquake should be recorded in that time span. Since then one M5+ event has already occurred - a M5.2 near Malatya on January 25, in an area that had a relatively high estimated probability of a M5+ earthquake. While the forecast highlights some areas as higher risk, the expected rate of aftershocks is fortunately quite low.
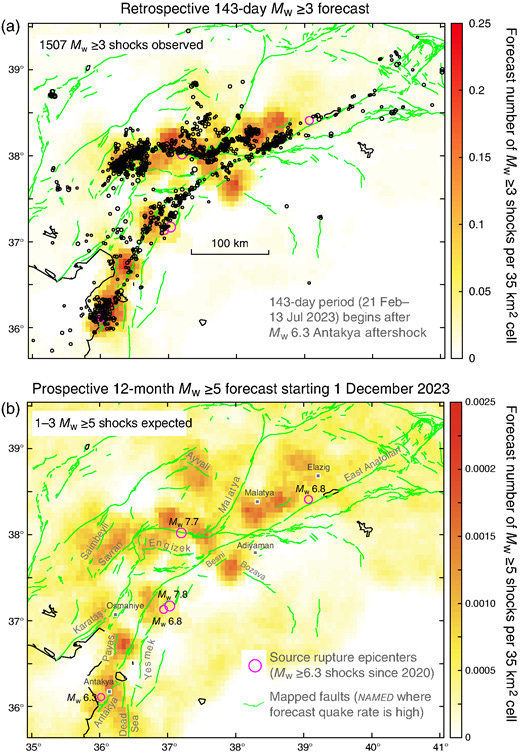
Impacts - Landslides, surface ruptures, and building damage
The Kahramanmaraş earthquakes produced wide ranging surface effects, including surface faulting, landslides, rockfalls, liquefaction, hydrological changes, building damage, and disruption of critical infrastructure. Some truly phenomenal events occurred, which have now been documented by field observations and remote sensing. Almost any images of these surface effects, if collected 40 years ago, would have been printed in textbooks ever since.
Jiannan Meng and colleagues made detailed maps of fresh surface ruptures using UAVs (unmapped aerial vehicle, or drone), documenting these fragile features before they could be eroded or modified. These observations provide accurate surface offsets that can be compared to remote sensing maps, and they provide insight into how the shallow parts of faults develop and are organized. Field observations of surface slip are very important, in part because geophysical models of fault slip often show a “shallow slip deficit,” where the upper few kilometers of the fault does not slip a much as the slightly deeper part. Thus, it is important to know what really happened at the surface. Exactly how this shallow slip deficit is made up over time remains a significant scientific question.
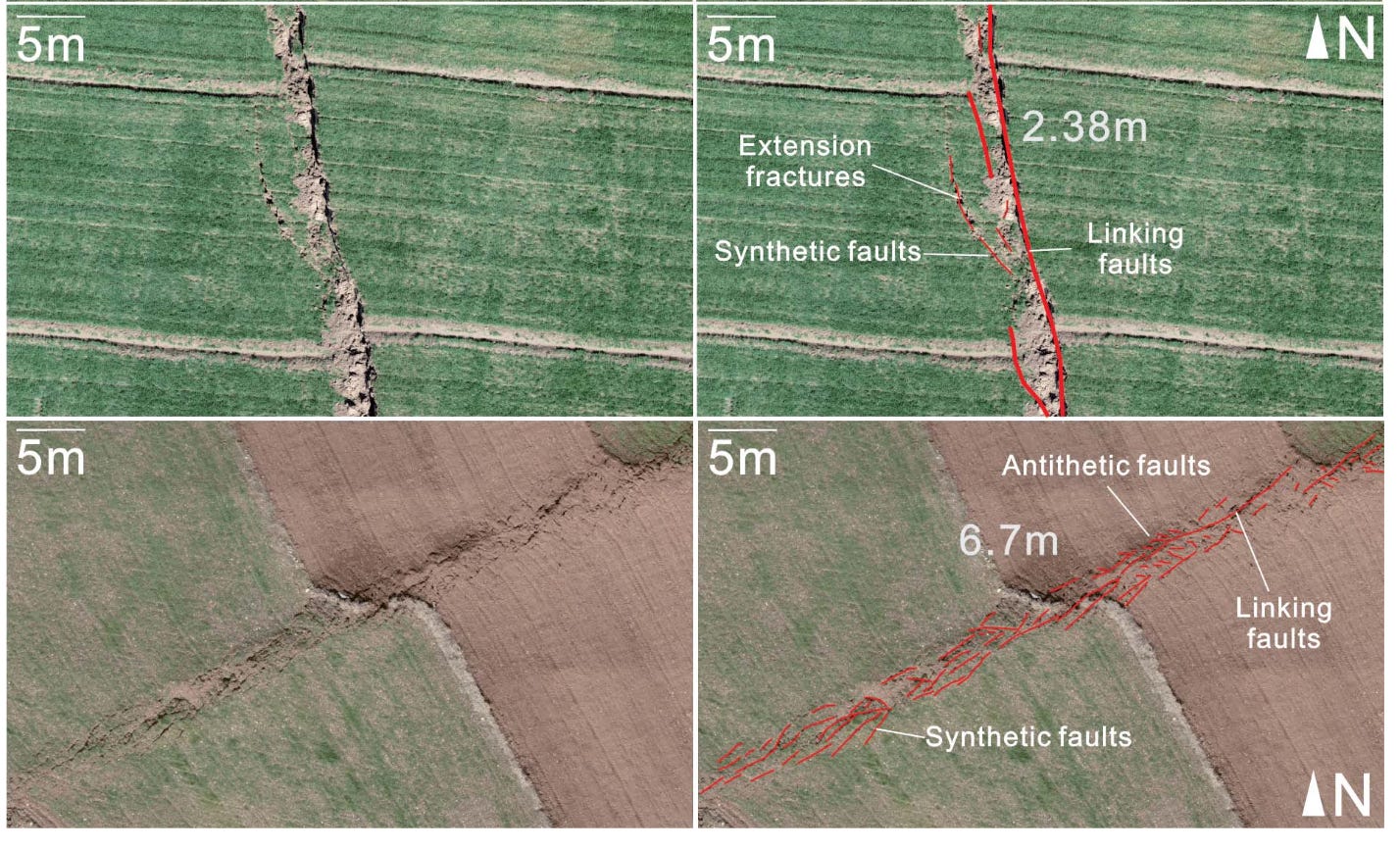
Tolga Görüm and colleagues documented a wide range of surface disturbances, including major landslides and rockfalls. They studied the widespread occurrence of these features, and linked their prevalence to local geology, climate zones, and the intensity of ground shaking. Some of the photographs in this paper are extremely compelling; it is interesting how the scars of previous landslides and rockfalls are often present in the areas of the most recent ground movements.
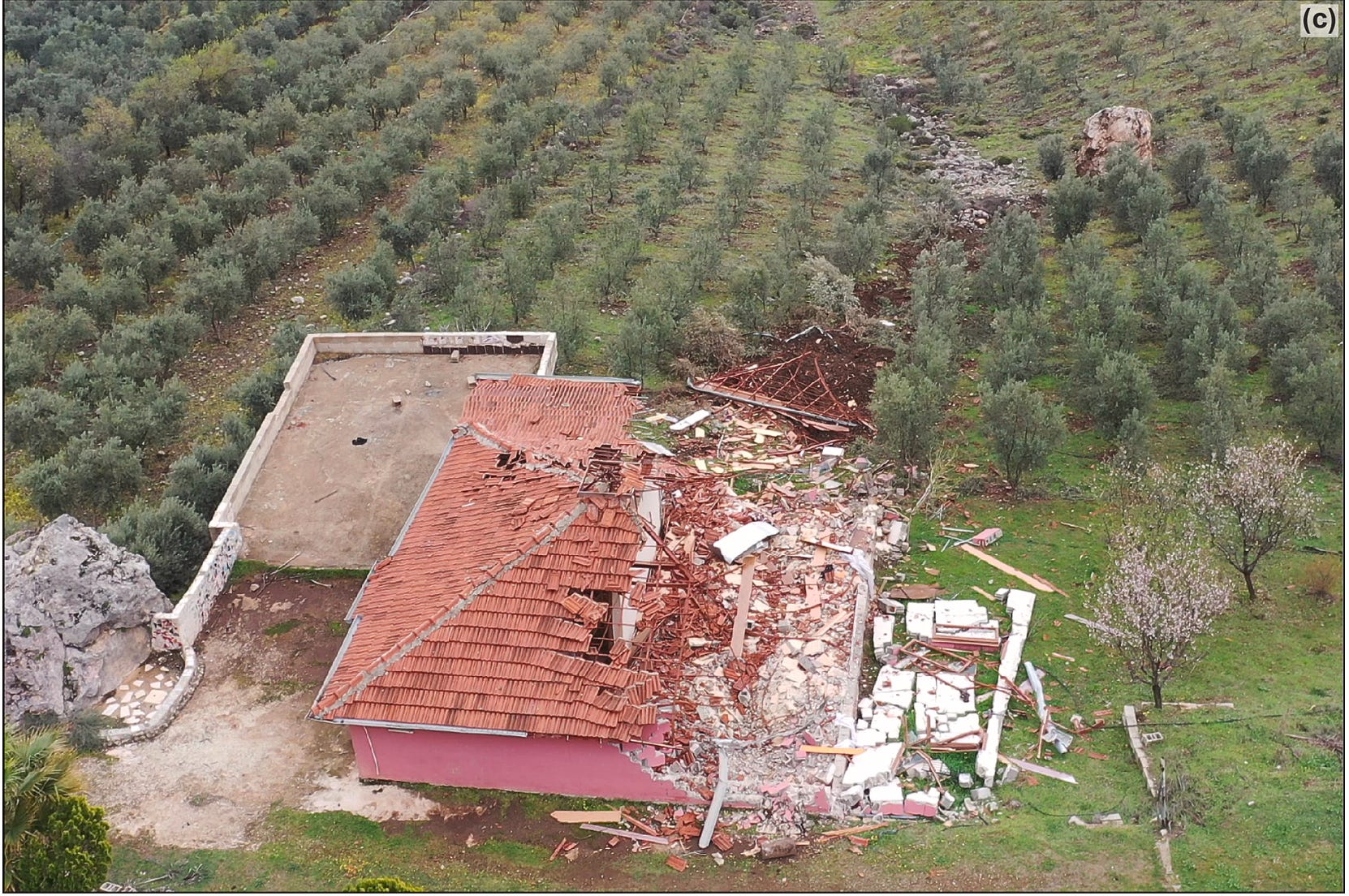
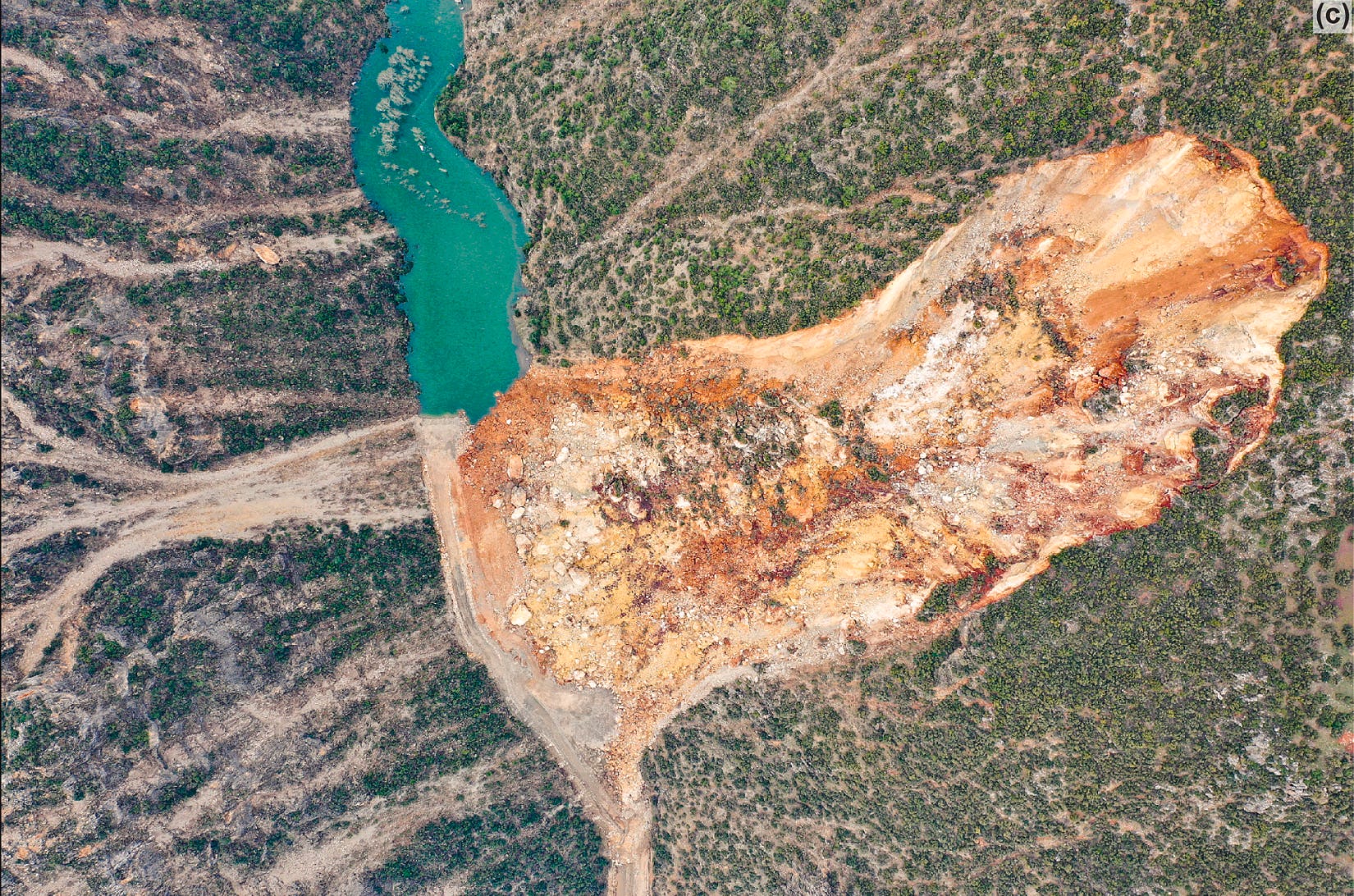
Gulen Ozkula and colleagues published a comprehensive study of ground effects, including detailed observations of damage to many different types of buildings. This paper is an excellent read for anyone interested in the thorny problem of building code design and enforcement, with many clear examples and good discussions of basic principles that are readable by non-experts like us. The authors compare the observed damage to observed ground accelerations, and discuss methods and effects of retrofitting.
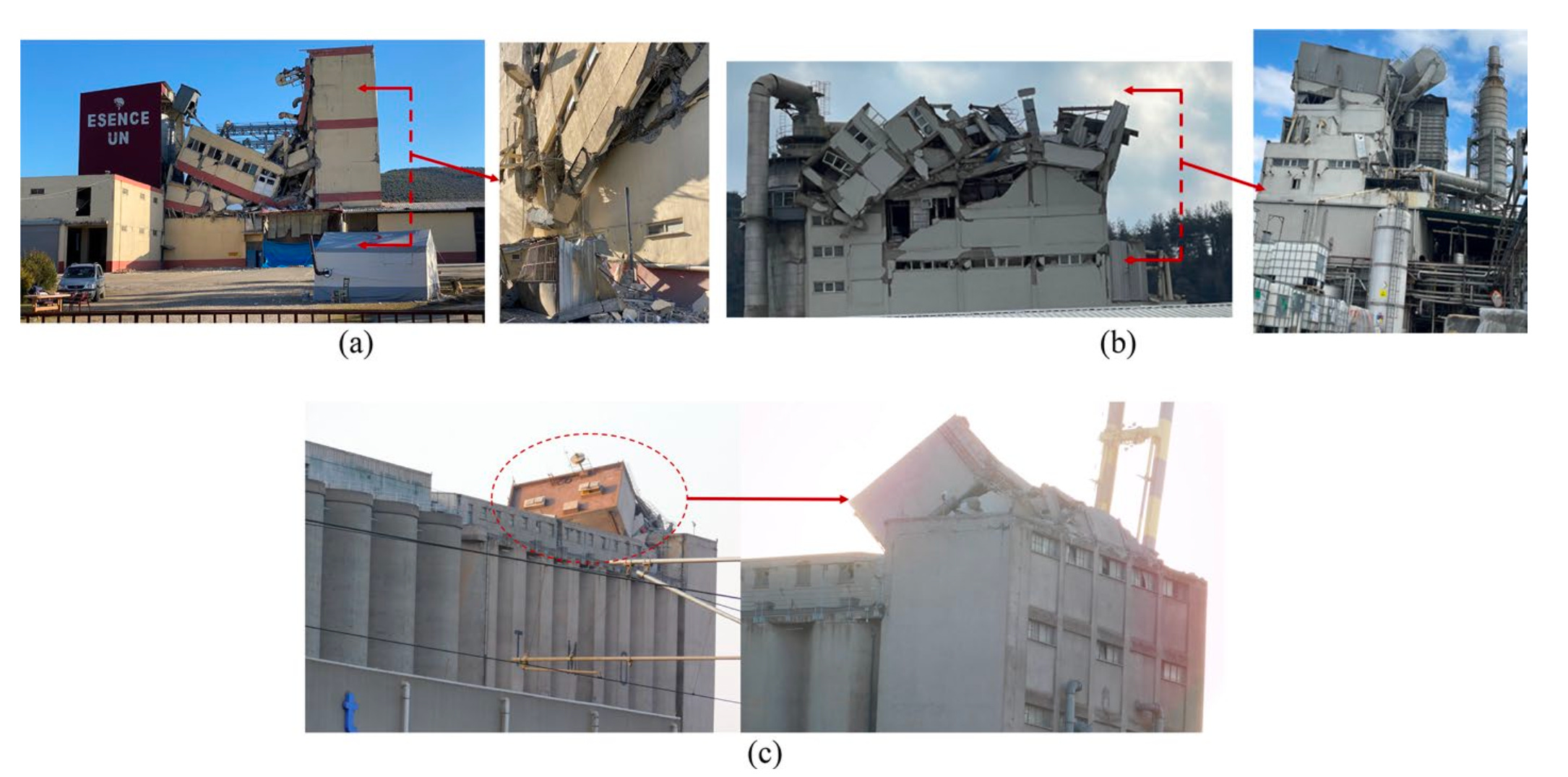
Ercan Işık studied the effects of strong shaking on mud-and-brick buildings, a extremely dangerous but common type of structure in rural areas in this region. While the standard (and easily given) advice is that people should build seismically resilient structures using wood, reinforced concrete or steel, that is clearly not a practical solution in many regions. Studies of the specific failure modes of adobe buildings can aid the development and implementation of practical methods for retrofitting or new construction. Notably, the insights gained from the 2023 Türkiye earthquake sequence can be applied to other seismically hazardous areas around the globe where adobe or unreinforced masonry structures are common - before a large earthquake strikes those areas.
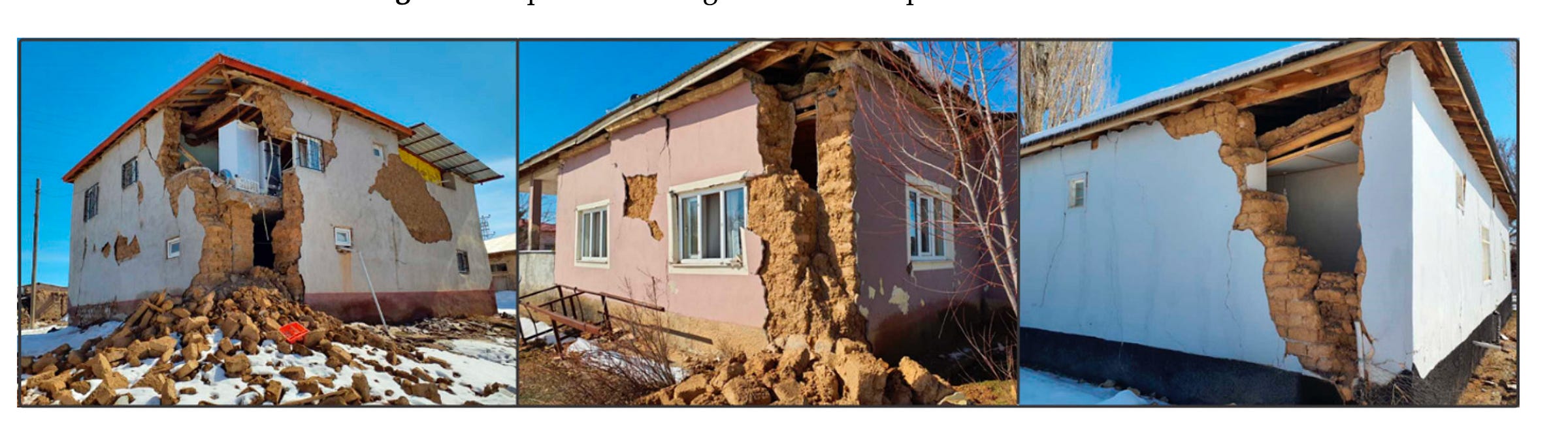
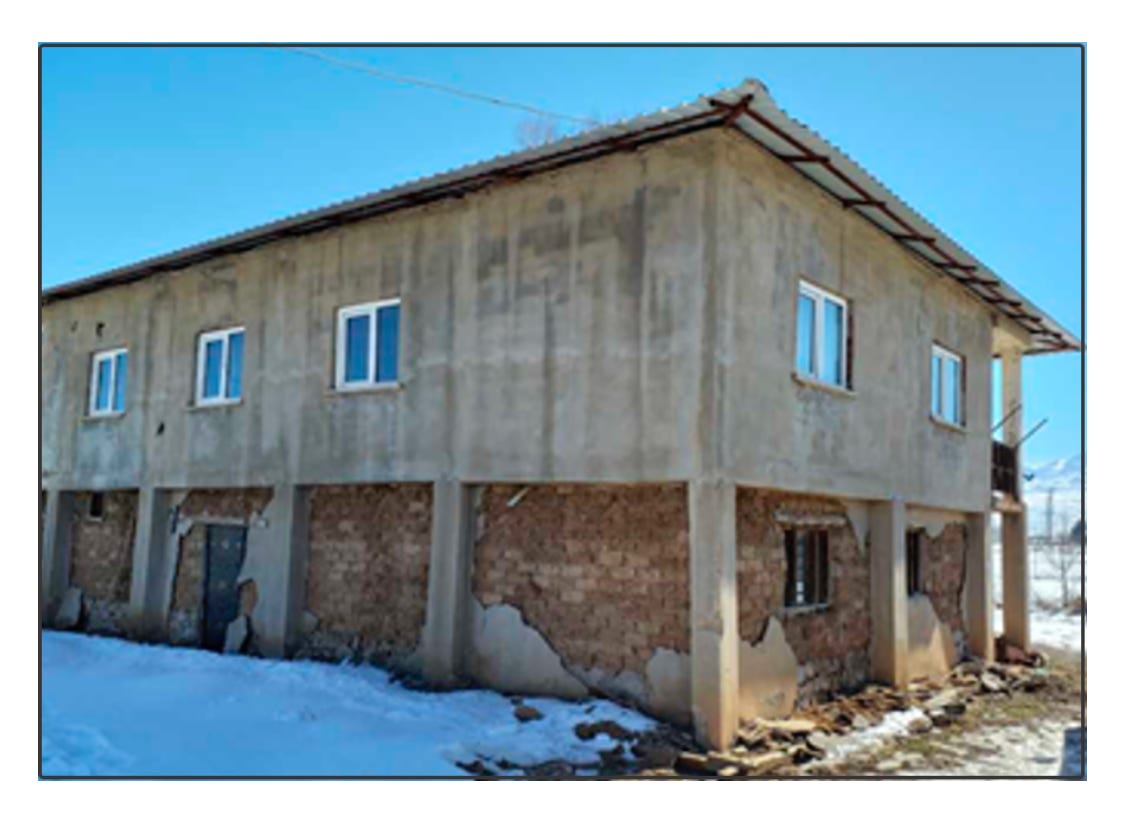
What happens after buildings are destroyed by an earthquake, and a huge cleanup has to begin? Spyridon Mavroulis and colleagues tracked the initial cleanup phase of some damaged urban areas using satellite images and field observations. They showed how the pressing need to deal with debris can lead to further problems, in the form of ad-hoc landfills that could pose continued threats to people and the environment for decades to come. This is a problem that we (the authors of this post) have never really considered, and which is not commonly discussed as a necessary aspect of earthquake preparation.
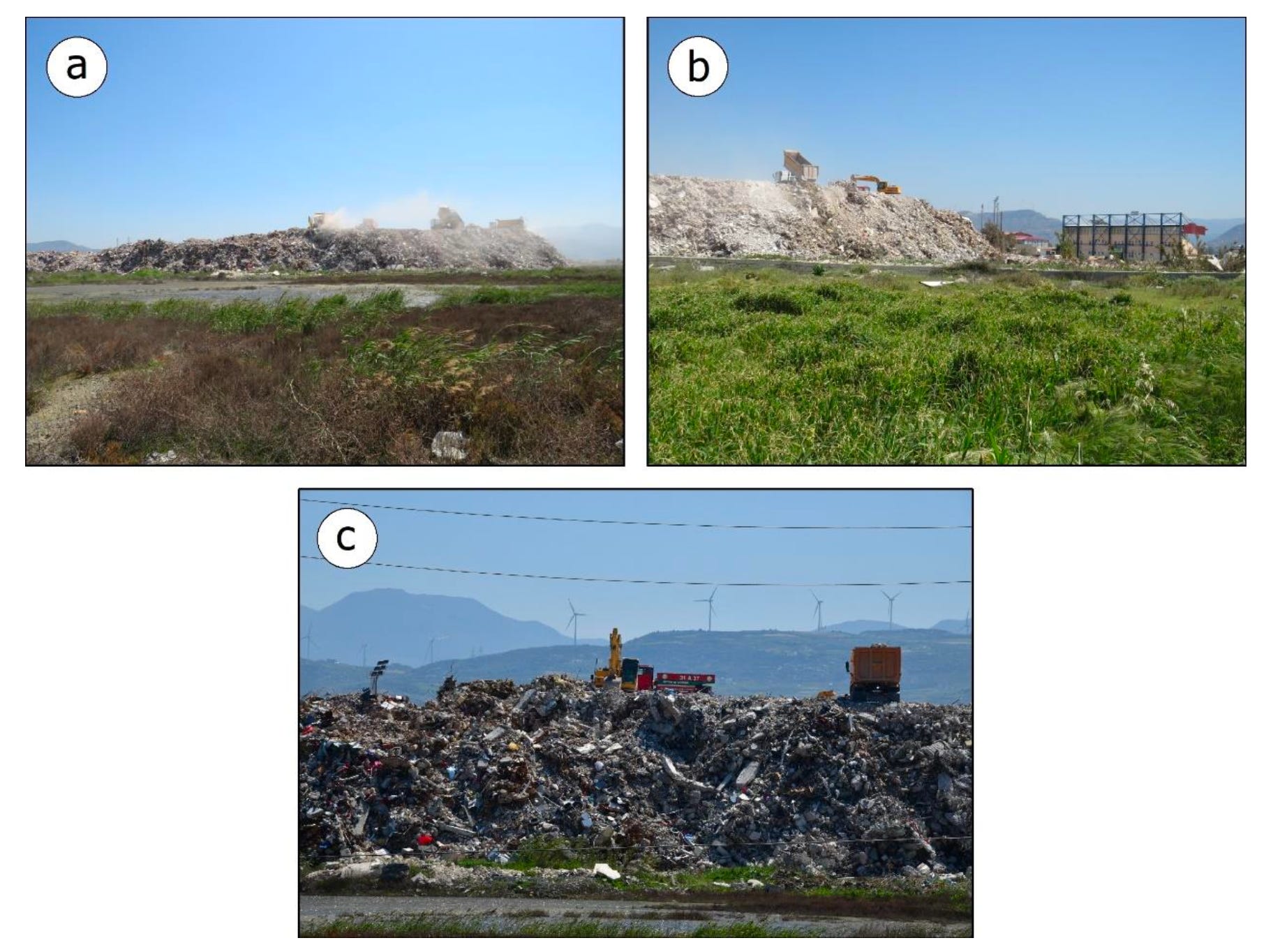
Precursory signals?
Scientists have tried for decades to identify “precursory” patterns before earthquakes that would allow them to effectively predict future ruptures - and have come up empty-handed. Many methods that appear to work for a few earthquakes, in one region, and in hindsight, often do not pan out for later events. However, every large earthquake is an opportunity to investigate this topic further and see whether previously proposed ideas work in practice.
Grzegorz Kwiatek and colleagues explore the patterns of seismicity during the lead-up to the Feb. 6 earthquakes. They find that the rate of seismicity was higher than normal twice during the nine years preceding the earthquakes - first in 2018, and then again starting mid-2022. They also observed a temporary decrease in the b-value, which measures the proportion of small earthquakes to large earthquakes, during mid-2022. Neither of these is out of the ordinary given the usual range of earthquake behaviors on faults.
What they did not find is more interesting. There is no evidence for any slow, aseismic slip on the fault (which has been widely proposed as a likely precursory behavior). They also did not observe any localization of seismicity, a process that was identified in the years prior to several large earthquakes in California and one in Turkey. And, although they looked for it, they were not able to identify any process of foreshocks cascading towards the epicenter - something that was observed in the 1999 M7.4 Izmit earthquake.
Ultimately, Kwiatek and his coauthors conclude - and we agree -
The variability of apparent earthquake nucleation processes observed for different events, the difficulty of distinguishing preparatory processes from other deformation transients that do not lead to major earthquakes, the participation of secondary faults, and an unknown false alarm rate, all suggest that with our current state of knowledge, intermediate-term earthquake warning—if possible—still lies in seismology’s future.
A path forward
Ezgi Karasözen and a group of Turkish early-career scientists published an article describing their vision for how to achieve seismic resilience in Türkiye. They propose widespread cultural changes, including universal geoscience education, specific preparation based on scenario modeling, establishment of local earthquake centers, and more effective science communication. Each one of these goals is admirable and attainable. However, the authors note that the number of students majoring in civil engineering, geological engineering, and geophysical engineering in Türkiye has dropped by more than half since a peak about 10 years ago - a trend that will need to change.
Over the last year, we have received emails from young students in Türkiye who want to know more about the causes of earthquakes and their associated hazards. Great earthquakes tend to resonate through society and science for decades. Each of the papers that we highlighted in this post should be viewed as a beginning of a conversation. So, our advice to those students is to take advantage of this moment and find a way into earthquake science, public policy, education, sustainable development, civil engineering, or any of the other myriad paths that can lead toward a better earthquake future.
Read our other posts about the Kahramanmaraş earthquakes. Many of them predate this Substack, which was inspired by those earthquakes and the public communication that followed. Our earliest posts can be found only on Twitter under @judithgeology. Some aspects of that earthquake communication are described in Gürer et al. (2023).
References:
Ben-Zion, Y. and Zaliapin, I., 2020. Localization and coalescence of seismicity before large earthquakes. Geophysical Journal International, 223(1), pp.561-583. https://doi.org/10.1093/gji/ggaa315
Di Giacomo, D., Storchak, D.A., Safronova, N., Ozgo, P., Harris, J., Verney, R. and Bondár, I., 2014. A New ISC Service: The Bibliography of Seismic Events, Seismol. Res. Lett., 85, 2, 354-360, https://doi.org/10.1785/0220130143
Görüm, T., Tanyas, H., Karabacak, F., Yılmaz, A., Girgin, S., Allstadt, K.E., Süzen, M.L. and Burgi, P., 2023. Preliminary documentation of coseismic ground failure triggered by the February 6, 2023 Türkiye earthquake sequence, Engng Geol., 327, 107315, DOI: 10.1016/j.enggeo.2023.107315
Gürer, D., Hubbard, J. and Bohon, W., 2023. Science on social media. Communications Earth & Environment, 4(1), p.148. https://doi.org/10.1038/s43247-023-00810-9
International Seismological Centre (2024), On-line Event Bibliography, event 625613033, https://doi.org/10.31905/EJ3B5LV6
Işık, E., 2023. Structural Failures of Adobe Buildings during the February 2023 Kahramanmaraş (Türkiye) Earthquakes, Applied Sciences, 13, 15, 8937, https://doi.org/10.3390/app13158937
Jia, Z., Jin, Z., Marchandon, M., Ulrich, T., Gabriel, A.A., Fan, W., Shearer, P., Zou, X., Rekoske, J., Bulut, F. and Garagon, A., 2023. The complex dynamics of the 2023 Kahramanmaraş, Turkey, M w 7.8-7.7 earthquake doublet. Science, 381(6661), pp.985-990. https://doi.org/10.1126/science.adi0685
Karasözen, E., Buyukakpinar, P., Ertuncay, D., Havazlı, E. and Oral, E., 2023. A call from early‑career Turkish scientists: seismic resilience is only feasible with “earthquake culture”. Seismica, 2(3). https://doi.org/10.26443/seismica.v2i3.1012
Kato, A. and Ben-Zion, Y., 2021. The generation of large earthquakes. Nature Reviews Earth & Environment, 2(1), pp.26-39. https://doi.org/10.1038/s43017-020-00108-w
Kwiatek, G., Martínez-Garzón, P., Becker, D., Dresen, G., Cotton, F., Beroza, G.C., Acarel, D., Ergintav, S. and Bohnhoff, M., 2023. Months-long seismicity transients preceding the 2023 MW 7.8 Kahramanmaraş earthquake, Türkiye. Nature Communications, 14(1), p.7534. https://doi.org/10.1038/s41467-023-42419-8
Mai, P.M., Aspiotis, T., Aquib, T.A., Cano, E.V., Castro‐Cruz, D., Espindola‐Carmona, A., Li, B., Li, X., Liu, J., Matrau, R. and Nobile, A., 2023. The Destructive Earthquake Doublet of 6 February 2023 in South‐Central Türkiye and Northwestern Syria: Initial Observations and Analyses. The Seismic Record, 3(2), pp.105-115. https://doi.org/10.1785/0320230007
Mavroulis, S., Argyropoulos, I., Vassilakis, E., Carydis, P. and Lekkas, E., 2023. Earthquake Environmental Effects and Building Properties Controlling Damage Caused by the 6 February 2023 Earthquakes in East Anatolia, Geosciences, 13, 10, 303, https://doi.org/10.3390/geosciences13100303
Melgar, D., Taymaz, T., Ganas, A., Crowell, B.W., Öcalan, T., Kahraman, M., Tsironi, V., Yolsal-Çevikbil, S., Valkaniotis, S., Irmak, T.S. and Eken, T., 2023. Sub-and super-shear ruptures during the 2023 Mw 7.8 and Mw 7.6 earthquake doublet in SE Türkiye. Seismica, 2 (3). https://doi.org/10.26443/seismica.v2i3.387
Meng, J., Kusky, T., Mooney, W.D., Bozkurt, E., Bodur, M.N. and Wang, L., 2024. Surface deformations of the 6 February 2023 earthquake sequence, eastern Türkiye, Science, 383, 6680, 298-305, DOI: https://doi.org/10.1126/science.adj3770
Ozkula, G., Dowell, R.K., Baser, T., Lin, J.-L., Numanoglu, O.A., Ilhan, O., Olgun, C.G., Huang, C.-W. and Uludag, T.D., 2023. Field reconnaissance and observations from the February 6, 2023, Turkey earthquake sequence, Natural Hazards, 119, 1, 663-700, DOI: https://doi.org/10.1007/s11069-023-06143-2
Ren, C., Wang, Z., Taymaz, T., Hu, N., Luo, H., Zhao, Z., Yue, H., Song, X., Shen, Z., Xu, H. and Geng, J., 2024. Supershear triggering and cascading fault ruptures of the 2023 Kahramanmaraş, Türkiye, earthquake doublet. Science, 383(6680), pp.305-311. https://doi.org/10.1126/science.adi1519
Toda, S. and Stein, R.S., The Role of Stress Transfer in Rupture Nucleation and Inhibition in the 2023 Kahramanmaraş, Türkiye, Sequence, and a One-Year Earthquake Forecast. Seismological Research Letters, 95 (2A), https://doi.org/10.1785/0220230252
Zelenin, E., Bachmanov, D., Garipova, S., Trifonov, V. and Kozhurin, A., 2022. The Active Faults of Eurasia Database (AFEAD): the ontology and design behind the continental-scale dataset. Earth System Science Data, 14(10), pp.4489-4503. https://doi.org/10.5194/essd-14-4489-2022