New paper suggests earthquakes on the southern San Andreas caused by lake filling - but is the evidence solid?
A study in 2011 came to the opposite conclusion. Let's take a look at the gritty details!
Citation: Hubbard, J. and Bradley, K., 2023. New paper suggests earthquakes on the southern San Andreas caused by lake filling - but is the evidence solid?. Earthquake Insights, https://doi.org/10.62481/a41ae25f
If this post has reverted to behind a paywall, download a pdf here. All of our analyses of recent research papers are intended to be permanently free to read and comment to allow open discussion by the scientific community.
A fascinating new paper published in Nature by Ryley Hill and others suggests that Lake Cahuilla, a prehistoric lake that has at various times swamped the Coachella and Imperial Valleys, plays an important role in deciding when the largest earthquakes occur on the southernmost San Andreas Fault. Many believe that this part of the fault - extending from the Salton Sea north towards Los Angeles - poses the greatest hazard, due to its long quiescence: although the average time between large earthquakes is estimated to be ~180 years, the fault has not slipped in a major quake in about 300 years.
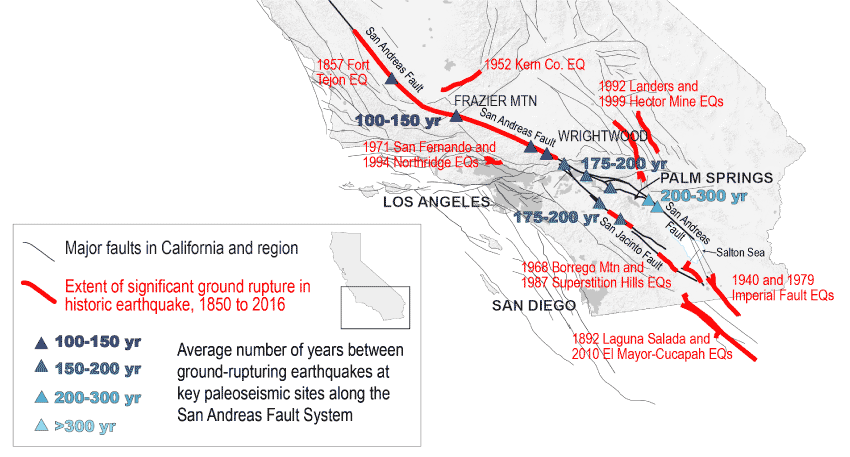
The Imperial and Coachella valleys lie just west the apex of the Colorado River Delta, which currently drains to the Gulf of California. Like all deltas, this is a distributary system: the river shifts over time between different courses. A sudden shift in water flow is termed an “avulsion.” When the Colorado River chooses a southern route, it flows directly to the Gulf of California, bypassing the Coachella and Imperial Valleys. Sometimes, however, it turns west. When this happens, the valleys - parts of which lie below sea level - must fill with water before the excess can spill southward. And thus, Lake Cahuilla is born, only to dry again when the rain does not fall or the river turns back to the south.
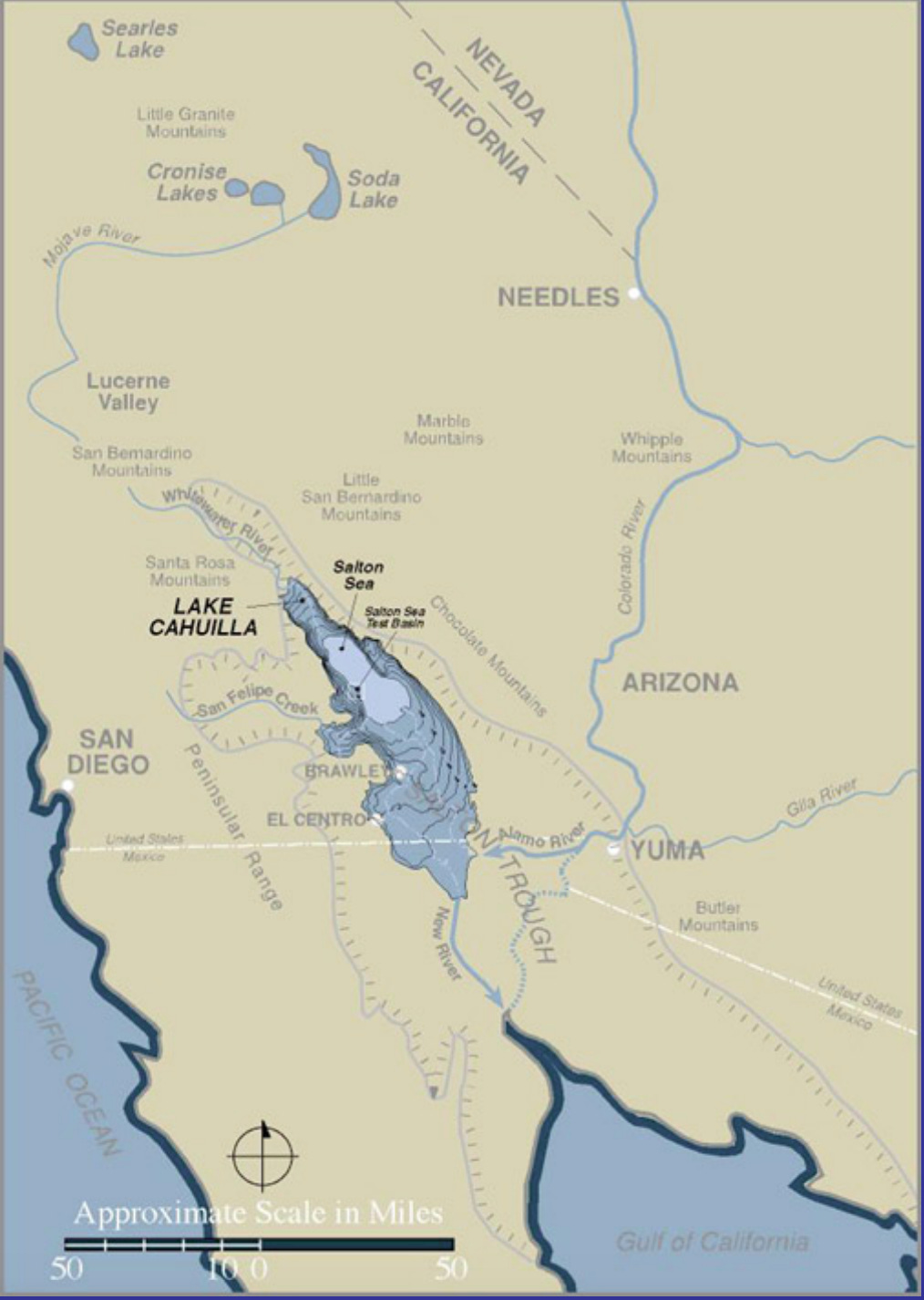
The authors of the new Nature paper compare the timing of highstands of ancient Lake Cahuilla with the geological record of large earthquakes - and find that they line up nicely. As shown in the figure below, this result “strongly suggests that all lake-filling events were accompanied by large earthquakes.” Further, they argue that the filling of the lake itself could have triggered the earthquakes by modifying the stress on the fault, and they use numerical modeling to make a compelling case that the relatively small effects of the lake could in fact control earthquake nucleation. This suggests that the long gap since the most recent earthquake can be explained by the dryness of the lake, and the authors warn that filling the lake now could be dangerous.
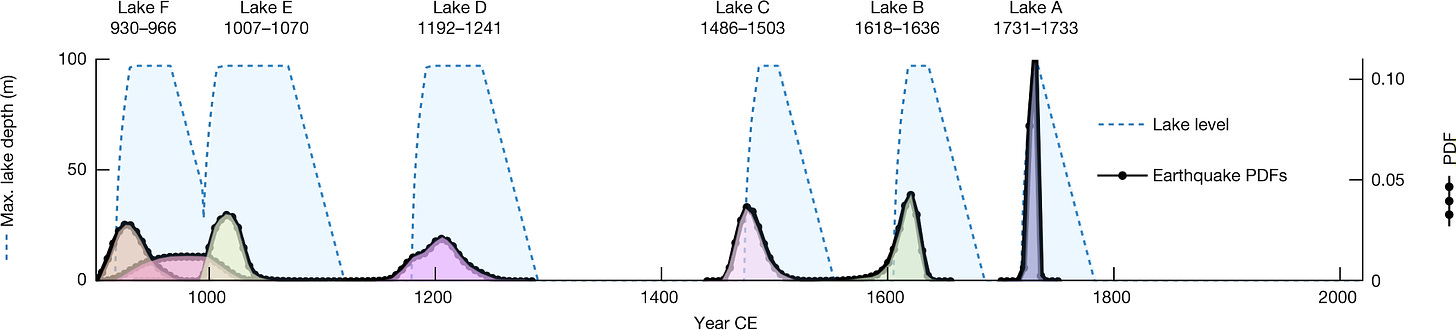
Given the major implications of the study, we decided to read the relevant papers carefully to better understand how the causation (lake filling induces earthquakes) is established.
The challenge of comparing earthquakes and lakes
Earthquake records on the San Andreas Fault are built from careful trenching work: pits dug along the fault. Within these pits, researchers carefully map the stratigraphic layers, identifying which layers were offset by fault slip and which were undisturbed, and looking for signs of earthquake disturbance like liquefaction. They then search the layers for material that can be dated - charcoal, bits of wood, seeds - and use these to develop a timeline of earthquakes at that site. By linking up earthquake slip from one trench to another, they can define an earthquake history that extends more than 1,000 years. This is a difficult discipline because a fault zone typically hosts multiple earthquakes, and disentangling their effects on the rocks is not easy. Furthermore, comparison between trench sites is difficult as the stratigraphy is inevitably different.
Understanding the timing of older lake-filling episodes is also hard, since the area filled was the same, time after time - newer lakeshores imprinted over older ones. A paper published in 2022 by Thomas K. Rockwell and others presented the most up-to-date vision of the lake history, inferring seven separate highstands from carbon-14 dates of hundreds of samples of charcoal, wood, and seeds that grew mostly on the dry lakebed and were washed up along the edges of the past lakes. There is actually significant overlap between these studies, as the paleoseismic trenches provide the best records of lake level over time.
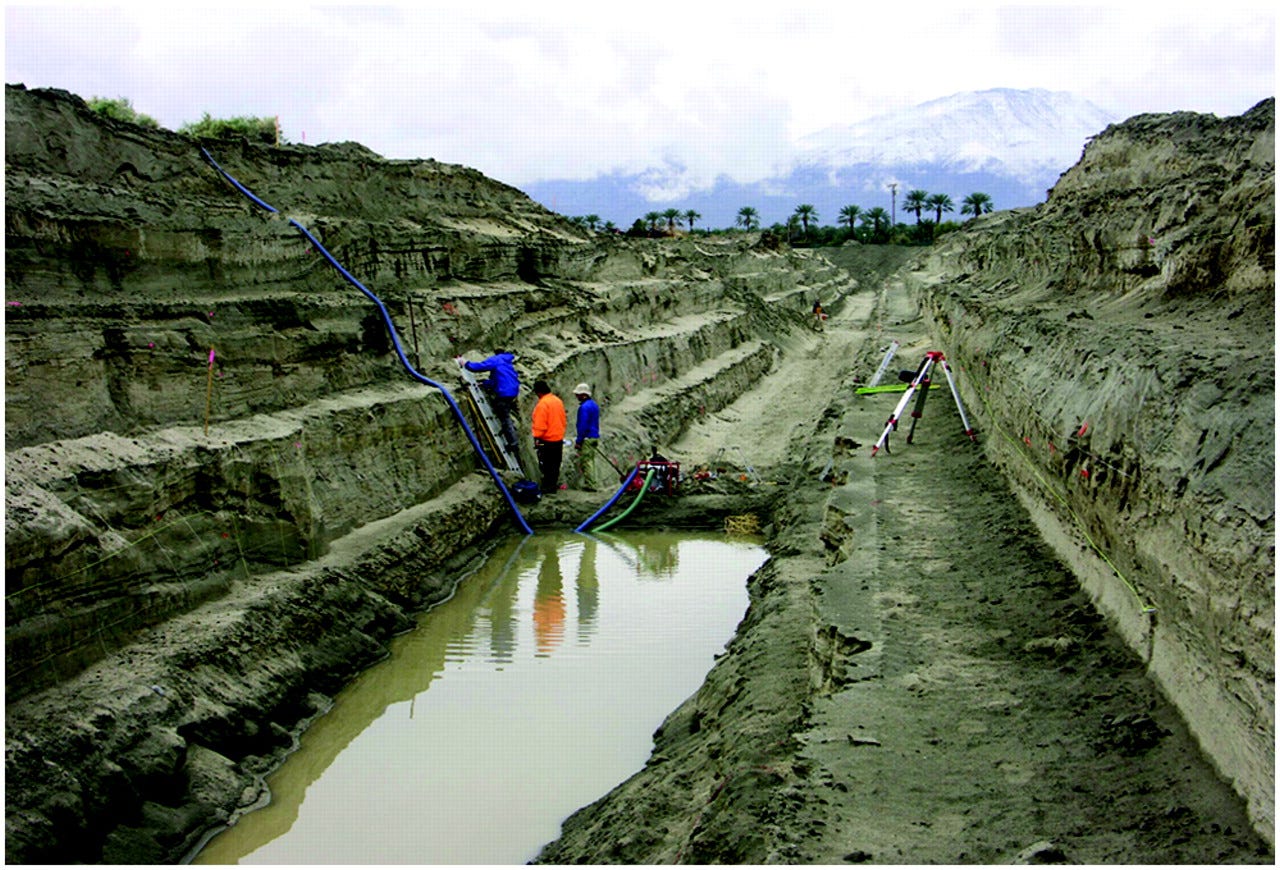
The paleoseismic records seem to show that the southern San Andreas produces earthquakes semi-regularly, spaced by decades to hundreds of years. The average time between large earthquakes is about 180 years, but the most recent quake was nearly 300 years ago, making this part of the fault one of particular concern - other parts of the San Andreas have ruptured more recently.
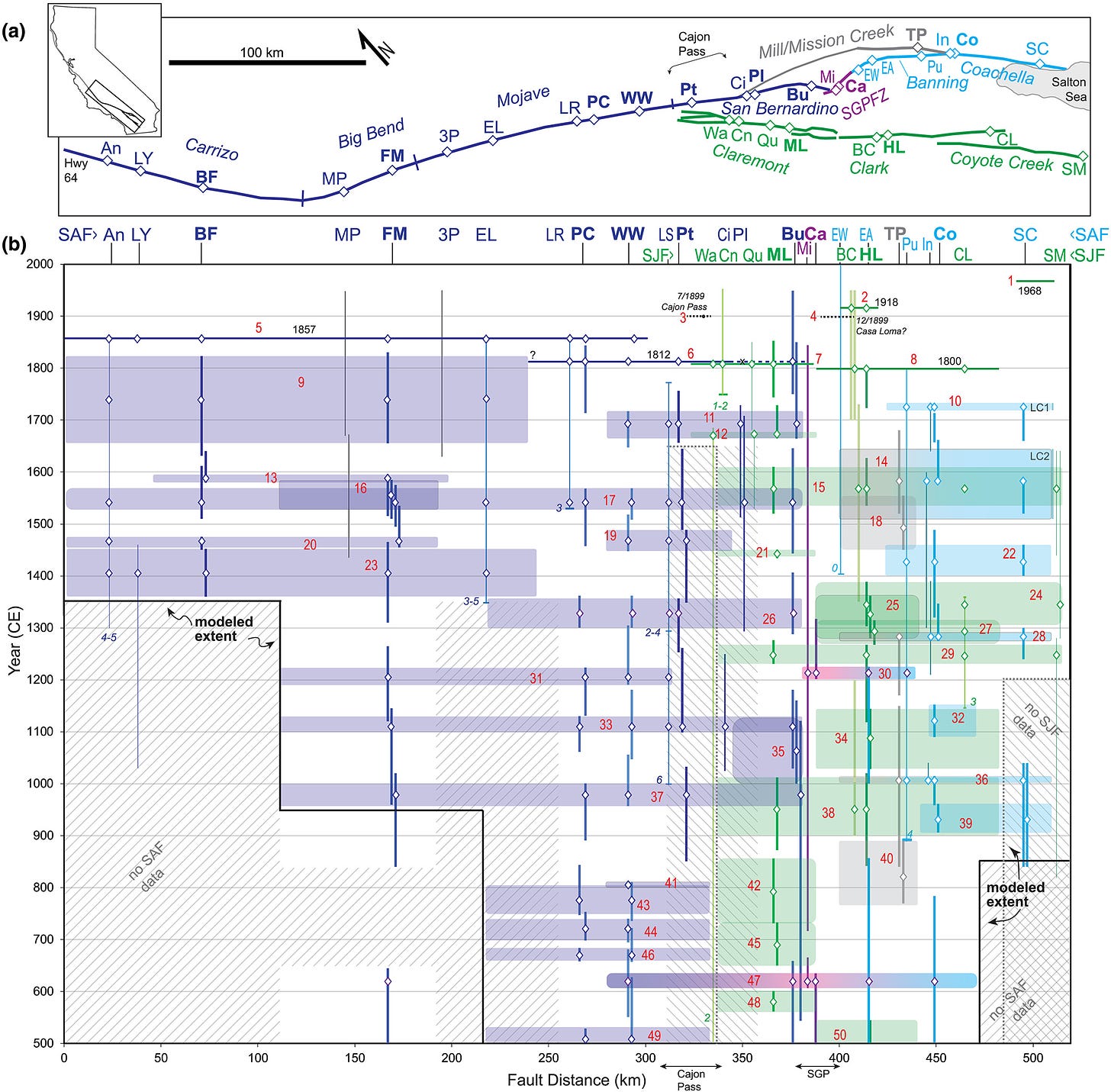
A link between Lake Cahuilla and earthquakes?
Interestingly, this is not the first time that a potential relationship between Lake Cahuilla and San Andreas earthquakes has been explored - several prior studies already exist, which reached conflicting conclusions. In 2011, a paper by Daniel Brothers and others used a geophysical technique called CHIRP to generate images of the stratigraphy below the Salton Sea, across faults that link together segments of the San Andreas north and south of the Salton Sea. Based on their results, they note that “at least one of the last three dry-basin floods coincides with an earthquake on the [Southern San Andreas Fault.]” They suggest that flooding of the lake could cause these linked faults to slip, potentially triggering large earthquakes on the San Andreas, and that the absence of flooding in more recent centuries could have “contributed to the prolonged interseismic period.”
Also in 2011, Belle Philibosian and others published a paper evaluating the timings of earthquakes and lake levels based on careful trenching; in their chronology, “the earthquakes do not tend to occur at any particular stage in the lake cycle.” One earthquake, they said, occurred as the lake was shrinking. Another was during a dry period. Two happened just before the lake filled. “The only potential relationship that can be observed,” they write, “is that at least four out of the seven earthquakes occurred shortly prior to a time when the lake either receded or filled.” Because of this, they acknowledge, there might be a relationship between the two physical processes: “Either the earthquakes were triggered by the early stages of the filling or dessication process, or […] a more likely possibility[:] earthquakes have triggered shifts in the course of the Colorado River that led to the filling or emptying of Lake Cahuilla.”
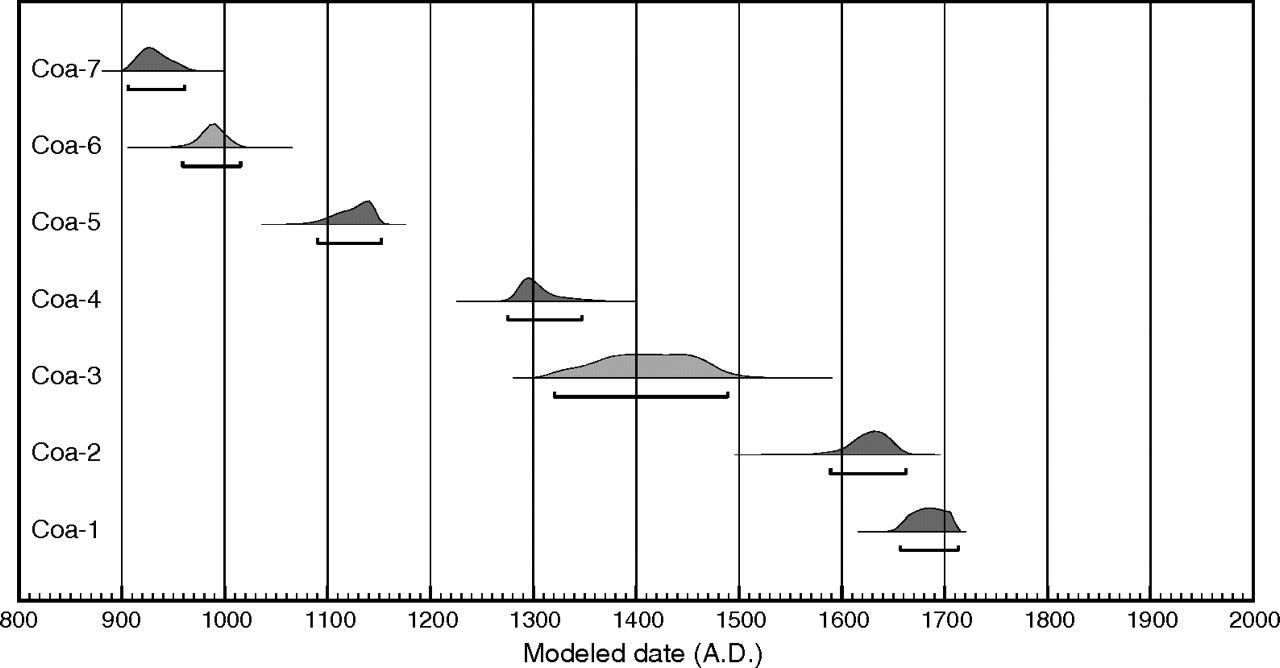
Although the conclusions of the recent Nature paper line up better with the CHIRP results of Brothers et al. (2011), the data used in the new paper is heavily drawn from the trenching work of Philibosian et al. (2011). How can two studies of the same phenomena, based in part on the same data, come to such different conclusions? Although some of the answer is new data, mostly it is re-interpretation: a different way of looking at the same observations.
How could the lake influence earthquakes, or vice-versa?
A lake sits on the Earth’s surface; earthquakes start far below. How could these two physical systems interact? Actually, that’s the least controversial part of the discussion - since there is no evidence of the details, all that has to be demonstrated is that a link is possible.
Lakes can trigger seismicity in two key ways: First, the weight of the water changes the stress on the fault below. Although in most places, strike-slip faults are vertical and therefore only slightly affected by vertical loads like gravity, in this area the fault is at an angle, about 30 degrees off of vertical. Second, fluids may be able to percolate along the fault and increase the fluid pressure on the fault surface, a process that is known to make faults more likely to slip. Hill et al. calculate the impact of some of these changes and show that they might be able to trigger earthquakes.
On the other hand, large earthquakes could also influence lake formation. Philibosian et al. note that shaking from an earthquake could cause local liquefaction or landsliding, blocking a channel or removing a levy, allowing the river to change course. This earthquake-induced avulsion process could lead to either filling or draining of the lake.
What are the differences in interpretation?
The two papers present not just different conclusions, but different fundamental chronologies. In the two plots above, the earthquake timings do not match: Philibosian et al. show two earthquakes that likely occurred between 1280 and 1500 CE; the new Hill et al. chronology shows no earthquakes during this time - they interpret that those two events were one and the same, and occurred later. More comparison shows further differences. For instance, for the most recent earthquake (Coa-1), according to Philibosian et al., occurred between 1657 and 1713 CE. Hill et al. revise this date range to between 1721 and 1731 - a significant difference, especially given that Hill et al. suggest that the lake highstand occurred from 1731 to 1733.
These differences led one reviewer of the new paper to write: “It is hard not to conclude that the interpretation of the trench data is fairly subjective and generally poorly unconstrained. […] I believe the authors did their best to carefully re-evaluate timing of events but it does seem open to bias given the difficulty interpreting the trench sections.” The authors, in their response to the above comment from a reviewer, write that their dates are different because they incorporate many more observational constraints that constrain the lake ages (i.e. the 2022 paper mentioned above; the author of that paper is a coauthor of the new study), which impact earthquake ages.
Fundamentally, though, the uncertainties in carbon-14 data are simply too large to allow a compelling comparison of earthquakes to lake level. Even the narrower range of dates above for Coa-1 does not demonstrate that the earthquake was triggered by lake filling. It just isn’t currently possible to use carbon-14 from mainly detrital sources, which may persist in the environment for years or even decades prior to deposition, to conclusively state whether an earthquake occurred just before or just after a lake filling event; the uncertainty ranges of the two events necessarily overlap.
Furthermore, the radiocarbon age estimates for the earthquakes are only valid IF the earthquakes actually occurred! Reading past earthquakes from trench walls is very tricky indeed, especially when multiple ruptures have affected a site. While Philibosian et al. (2011) identified up to eight potential earthquake events in their trenches, they clearly noted that several of these are only weakly supported by the available field relationships, and those relationships could represent effects of one or more of the other earthquakes. The main issue is that very few observable structures are truly diagnostic of an earthquake taking place at a specific moment (stratigraphic horizon); a preponderance of evidence is needed to identify the more cryptic events.
Ultimately, the comparison of earthquakes and lakes requires relative dating using geological cross-cutting relationships. If a trench has many layers exposed, some of those layers may reflect lake filling events. If there are fault offsets that terminate just below or just above those lacustrine layers, then it is possible to tell the relative timing of the earthquakes and the lakes, even if you can’t know exactly when the sequence happened; these interpretations are the actual constraints of interest in these papers.
For this, Hill et al. (2023) revisit the observations of Philibosian et al. (2011), with a few key ideas that significantly change the relative dating interpretations.
1. Are organic-rich layers soil? Or are they rafts of wood and charcoal carried by a rising lake?
Philibosian et al. (2011) observed several organic-rich layers in their trench stratigraphy, which they interpreted as soils exposed to the air or possibly in-situ burn areas. Hill et al. instead interpret these as rafts of organic materials: the organics, they say, grew within the basin when it was dry. When the lake filled, it floated bits of wood and charcoal and left them along the shore as a kind of biological mat.
With this model in mind, they look at an earthquake identified by Philibosian et al. interpreted to have occurred between 1090 and 1152 CE, named “Coa-5” (location = Coachella, 5th earthquake from present). This quake folded sediments, including at the top of the folded section, a layer rich in organics. Above the folded layer are undeformed clays and silts: lake sediments. According to Hill et al., this event must have occurred just after the area was flooded by a rising lake, allowing deposition of the “raft” but before the lake had a chance to deposit fine sediments. Philibosian et al. instead interpret that the earthquake occurred before flooding: the folded layer is a soil exposed to the air, they suggest, which was folded during the earthquake and later flooded by the rising lake. The difference of opinion - before flooding or just after - lies in the interpretation of the organic layer. Notably, there do not appear to be any lake silts (unit 4L, above the green line) involved in the folding. A similar line of argument is used for several other organic layers that Philibosian et al. (2011) interpreted as terrestrial, which have been reinterpreted as lacustrine.
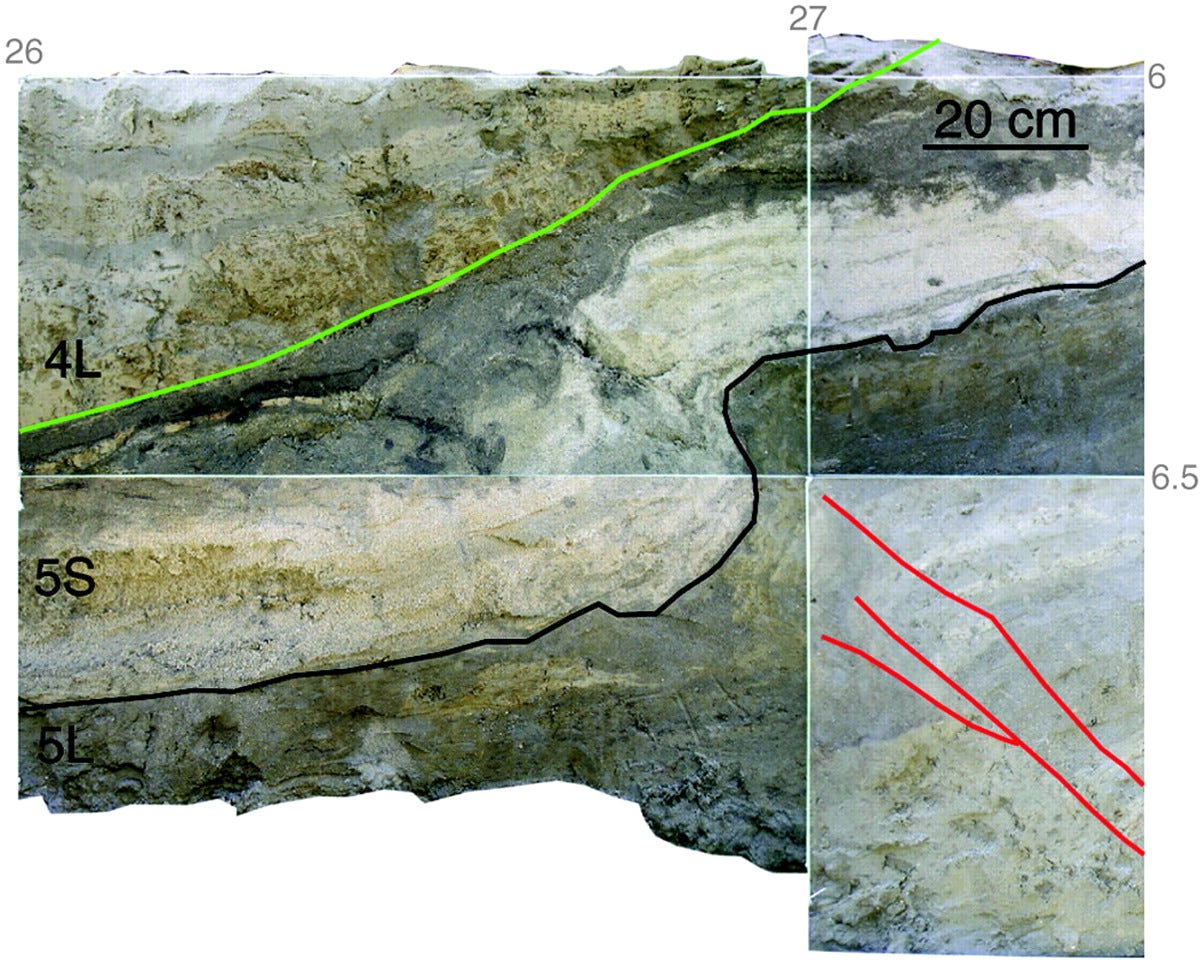
2. Do fine sand layers represent beach deposits or sand dunes?
The stratigraphy exposed in the Coachella paleoseismic trenches oscillates between fine-grained (silt and clay), laminated lake deposits and intervening coarser (sandy) deposits. Philibosian et al. (2011) interpret many of the sandy deposits as aeolian sands, representing wind-blown material derived mostly from the exposed lakebed (this kind of aeolian sand can be seen today swamping the eastern shoreline of Owens Lake, which dried up due to diversion of water into the Los Angeles aqueduct). Aeolian sands tend to have a very specific grain size range, and are typically laminated. In this interpretation, the sandy layers sit on top of erosional surfaces that postdate lake level fall. If a fault strand cuts the lake deposits but does not cut the sands above, then the earthquake could have occurred EITHER when the lake was full of water, or any time after the lake drained and before the sand dunes were deposited - there is a gap in time in the stratigraphy along the top of the lake sediments due to intervening erosion.
In contrast, Hill et al. (2023) follow Rockwell et al. (2018) and Rockwell et al. (2022), who re-interpreted many of these sandy deposits as recessional shoreline sands. (Thomas K. Rockwell, the first author of the 2018 and 2022 papers, is a coauthor of the Hill et al. paper) When lake level falls, the receding high energy beach zone can rework the lakebed sediments, winnowing out any fine material and leaving behind a thin deposit of well-sorted, stratified sands sitting directly on top of the older lake sediments. This is a classic type of deposit identified in regressive-transgressive sedimentary sequences globally. In this interpretation, there is virtually no time gap between the lake silts and the overlying sands, and any fault strands that cut the lake deposits but are buried by the shoreline sands must have occurred while the lake was nearly full.
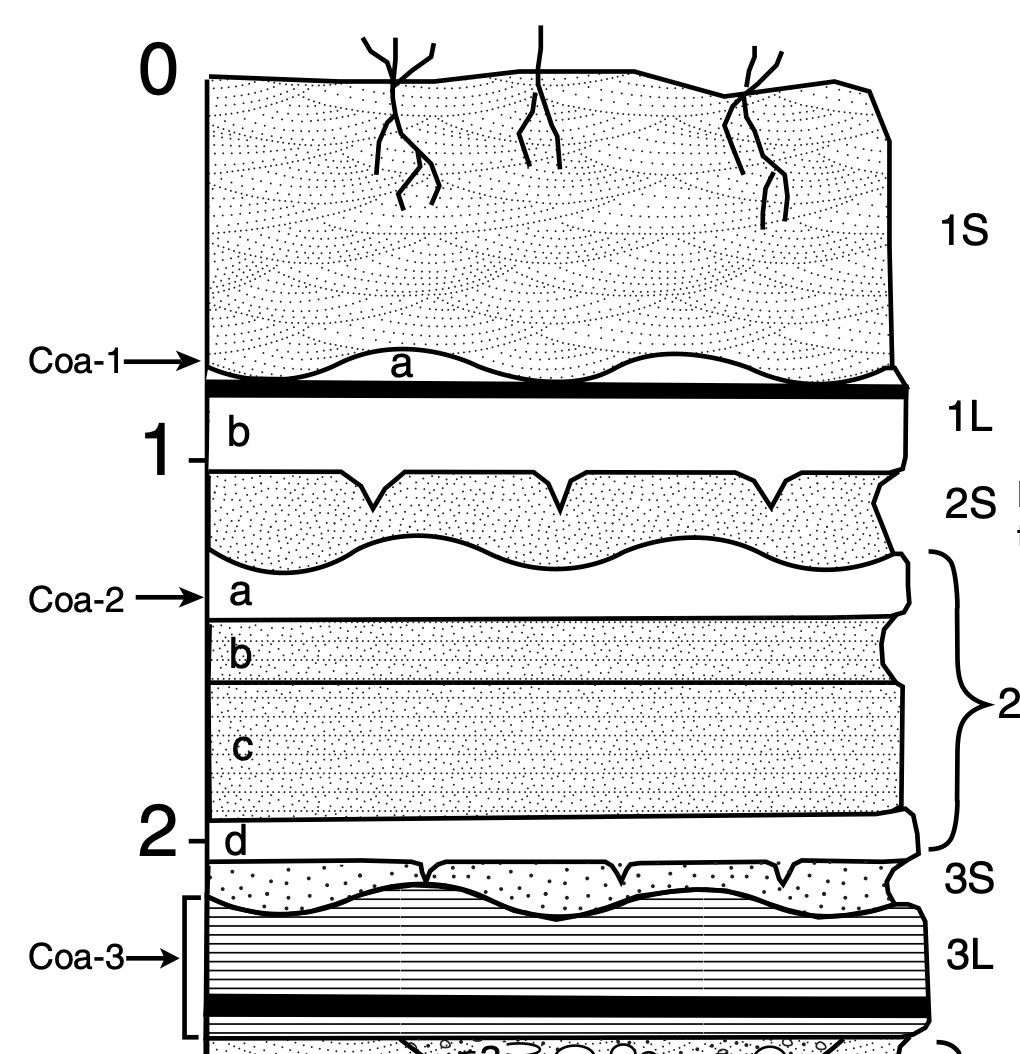
3. Does liquefaction require presence of lake water?
When water-saturated sediments are shaken by an earthquake, they can liquefy - lose their cohesive strength and deform like toothpaste. Research on earthquakes in this area - including both Hill et al. (2023) and Philibosian et al. (2011) - has assumed that this process can only happen when the soils are flooded by the lake. Therefore, any plastic sediment deformation seen in the trenches should be evidence for an earthquake that occurred when the lake was full.
This question - whether areas must actually be flooded in order to undergo seismically induced soft-sediment deformation - is particularly relevant for the most recent inferred earthquakes: Coa-1 and Coa-2. Both Hill et al. and Philibosian et al. both agree: these earthquakes occurred while the lake was full - because the sediments are plastically contorted, something that could only happen if they are wet. Hill et al. directly state that inferred earthquake Coa-2 “clearly occurs [while the lake was flooded], based on extensive liquefaction (only possible with water present).”
Notably, however, the deformed lake sediments used to identify Coa-1 flowed downhill and overrode sediments deposited after the lake receded (regardless of whether the 1S sand layer is aeolian or a recessional shoreline). Could groundwater expelled to the surface during a liquefaction event have promoted the soft-sediment slumping? Or was it simply a wet season when the earthquake struck?
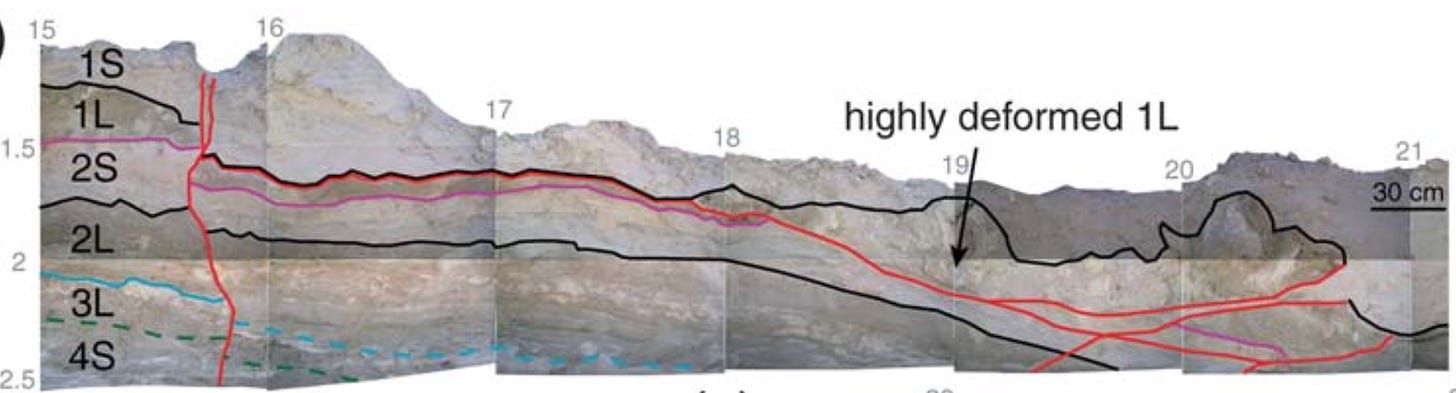
Inferred earthquake Coa-2 is also associated with impressive soft-sediment deformation features. While it is easy to imagine that the highly contorted sediments might represent a lakebed that was ‘stirred up’ by the shaking, liquefaction can actually only happen in buried sediments. These features are formed when excess pore pressure develops in a sandy bed due to porosity collapse - the rigid structure built by stacked sand grains fails due to shaking, and the fluids in the spaces between grains are suddenly compressed by the weight of overlying sediment. Such pressures can usually only be sustained if there is an overlying low-permeability layer, such as finer-grained material, which can hold the fluids in - indicating that the folded layer was already buried when the earthquake happened. So why couldn’t the pore water have been regular groundwater, rather than lake water?
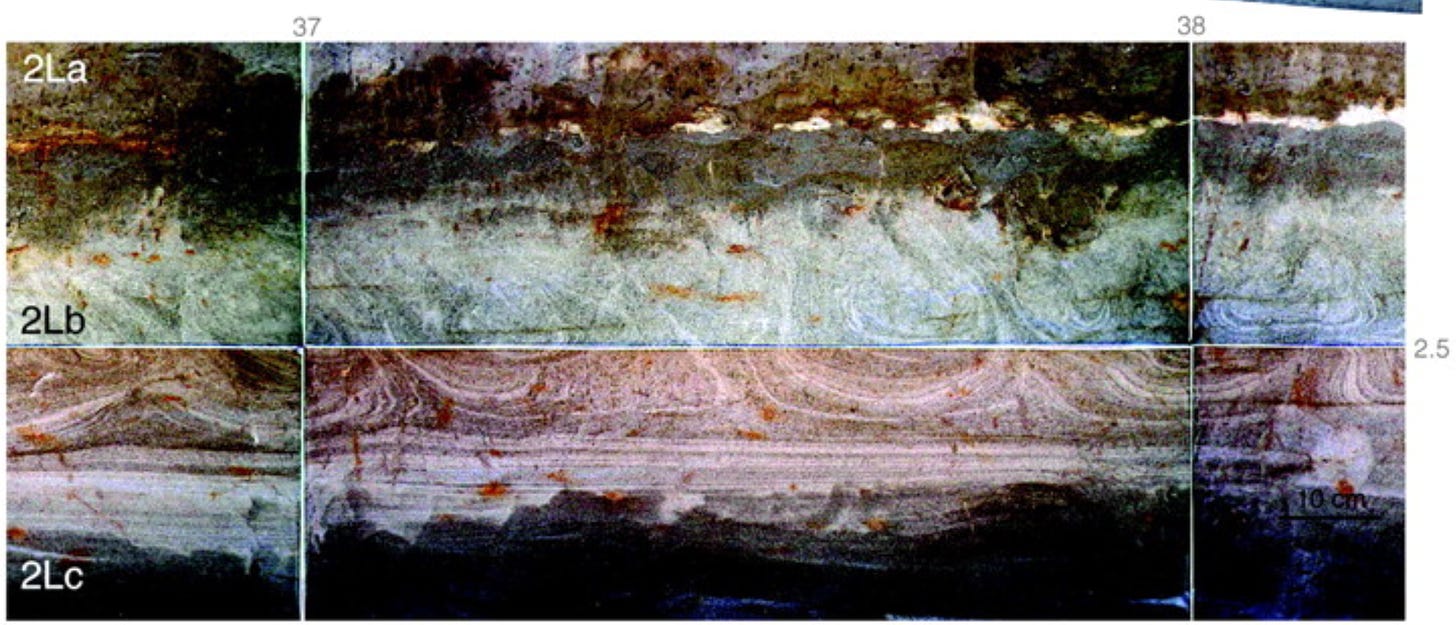
If the water table is shallow enough, the earthquake is strong enough, and the right types of sandy deposits exist in the subsurface, liquefaction can occur. Widespread earthquake-triggered liquefaction was observed in 2018 in Indonesia on the gentle slopes of an alluvial valley - no lake in sight, just layered, sandy sediments saturated by irrigation.
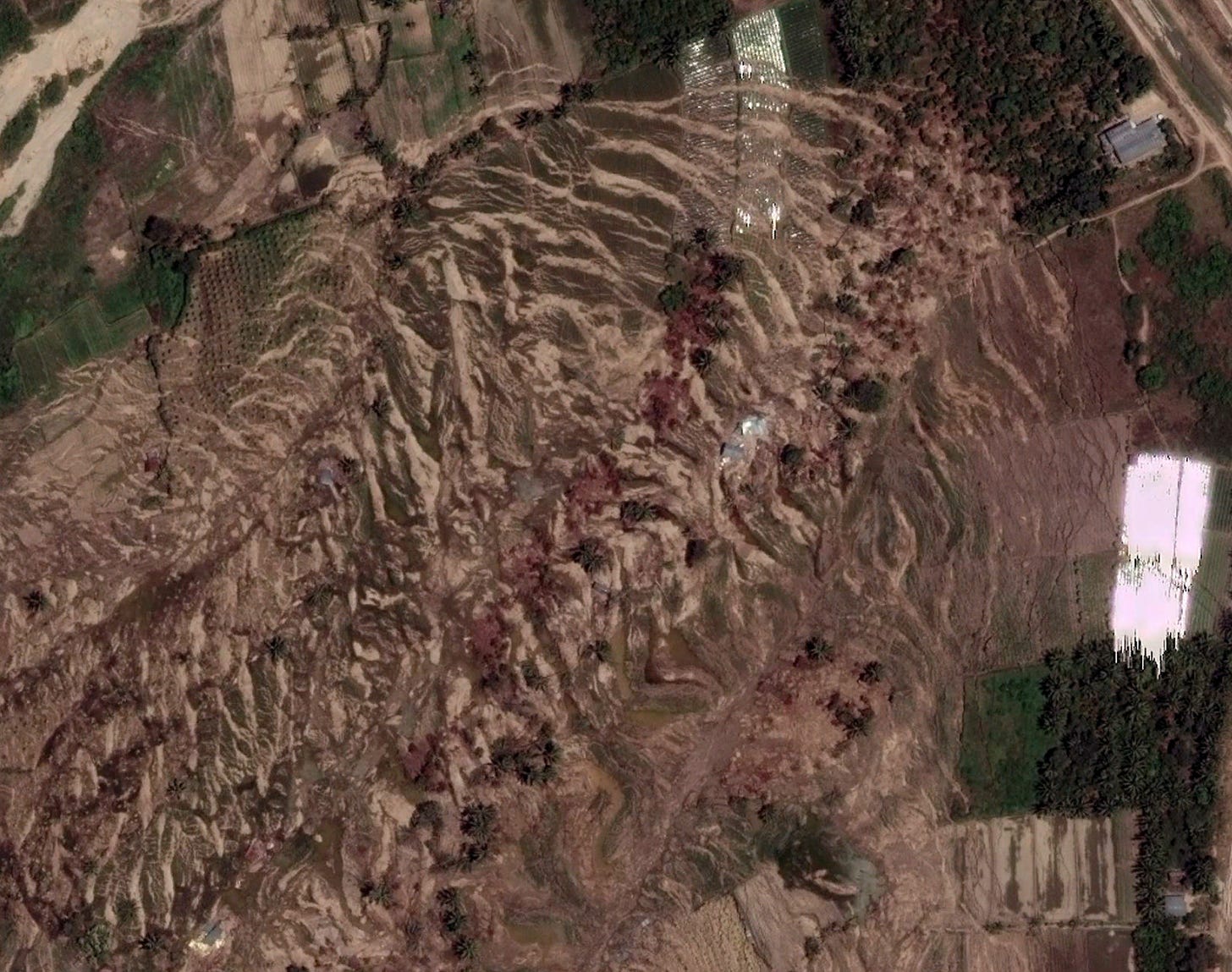
In fact, even today, official maps from Riverside County mark the dry areas as having shallow groundwater - with high to very high liquefaction susceptibility. The trench photo near the beginning of this article confirms that groundwater is not currently very deep (~6 meters) at the Coachella paleoseismic site; hydrological conditions in the past are even more uncertain. The interbedded lake and alluvial sediments in this area present a very interesting setting for shallow groundwater.
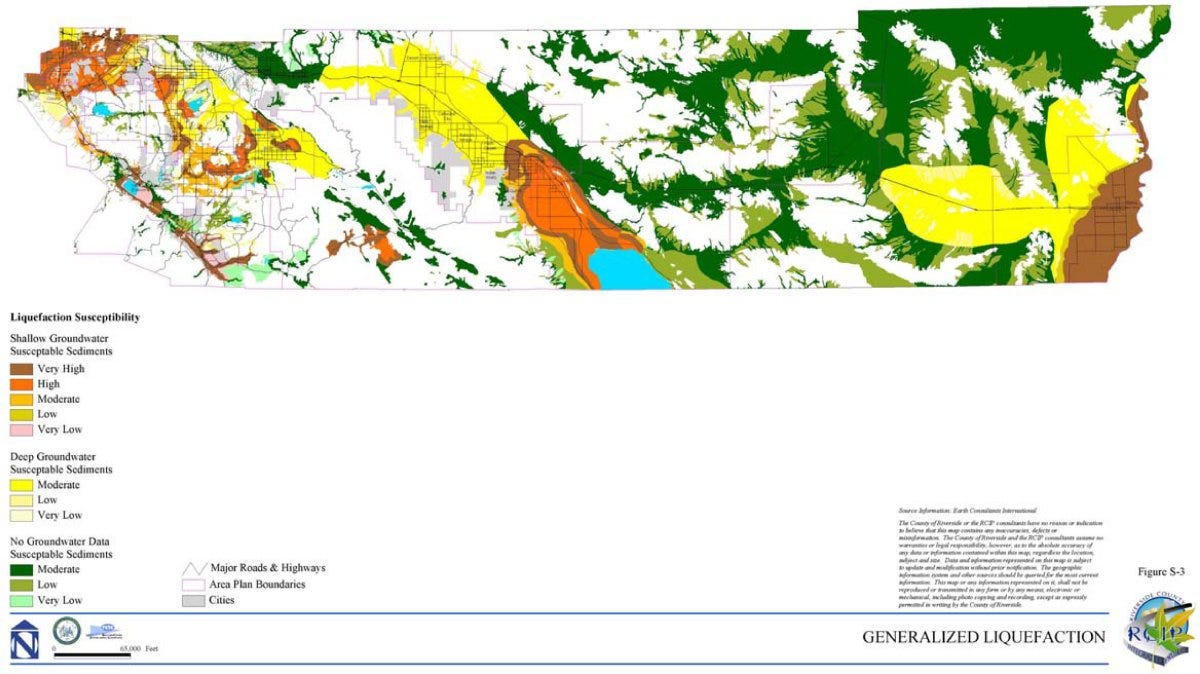
Both papers agree that one earthquake occurred during a dry period
What about that earthquake that - according to Philibosian et al. - occurred during a dry period? Actually, there Hill et al. (2023) agree: yes, earthquake Coa-7 occurred between two lakes. This quake was not related to lake filling. This earthquake is on both chronologies, although it is a little hard to see on the Hill et al. plot - a low-amplitude bulge colored in red just before 1000 CE, overlapping with two earthquakes on either side. That earthquake shows that the fault can slip without the influence of the lake, even when not much time has passed since a previous event.
Summing up:
Paleoseismology is a difficult science, requiring the careful accumulation of evidence, combined with “reading” of stratigraphic layers to tell a consistent story of ancient environments. The same data can sometimes allow multiple possible interpretations. These geological interpretations and re-interpretations are fundamental to the Hill et al. (2023) argument, although they do not make a very prominent appearance in their text. The critical stratigraphic assertions also do not seem to have been subjected to challenging review - yet.
Based on the information we have, it certainly seems possible that filling of ancient Lake Coachella triggered earthquakes. If the stratigraphic re-interpretations are correct, then this does seem like the most likely interpretation. But it also appears that the link between earthquakes and lake filling is not completely settled. Some of the previously identified earthquakes may not really exist. Further trenching is clearly needed to find better evidence and increase the identification of these events from “possible” to “probable”. Alternatively, if some events can be confidently excluded, then the recent quiet period might be not so unusually long. Notably, the fault is known to experience creep - slow, aseismic slip. Is it possible that some of the paleoseismic evidence of earthquakes may be caused by this slower kind of slip? Philibosian et al. (2011) actually identify such creep in their trench: fault strands break the youngest unit - deposited at a time recent enough to exclude earthquake behavior.
The stratigraphic interpretation of the sandy units and the organic layers also needs to be settled, preferably with further direct study of the units in the field. Are there characteristics of these different environments that can determine their terrestrial or underwater origin? Bioturbation (stirring up of the ground surface sediments by plant roots or burrowing animals) seems like a critical factor, as it should be common beneath aeolian sands but uncommon beneath recessional shoreline sands). Finally, the proposed link between liquefaction and plastic sediment deformation with subaqueous conditions needs to be tested; not only to unravel the lake-quake story, but because the Coachella and Imperial Valleys have liquefaction hazard even today.
(As a side note, published papers commonly cite Sieh et al. (1986) and Sieh and Williams (1990) as evidence for the last large earthquake occurring during the last lake highstand. Unfortunately, the first source is an AGU abstract that doesn’t mention the lake, and the second source provides no actual observations of the relevant field relationships of the highstand earthquake, instead focusing on other problems like measuring the fault slip rate. Perhaps the highstand bar site should be re-investigated?)
Papers cited:
Bradley, K., Mallick, R., Andikagumi, H., Hubbard, J., Meilianda, E., Switzer, A., Du, N., Brocard, G., Alfian, D., Benazir, B., Feng, G., Yun, S.-H., Majewski, J., Wei, S., Hill, E.M., 2019. Earth-triggered 2018 Palu Valley landslides enabled by wet rice cultivation. Nature Geoscience 12, 935-939, https://doi.org/10.1038/s41561-019-0444-1.
Brothers, D., Kilb, D., Luttrell, K., Driscoll, N., Kent, G., 2011. Loading of the San Andreas fault by flood-induced rupture of faults beneath the Salton Sea. Nature Geosci 4, 486–492. https://doi.org/10.1038/ngeo1184
Hill, R.G., Weingarten, M., Rockwell, T.K, Fialko, Y., 2023. Major southern San Andreas earthquakes modulated by lake-filling events. Nature. https://doi.org/10.1038/s41586-023-06058-9
Philibosian, B., Fumal, T. and Weldon, R., 2011. San Andreas fault earthquake chronology and Lake Cahuilla history at Coachella, California. Bulletin of the Seismological Society of America, 101(1), pp.13-38. https://doi.org/10.1785/0120100050.
Rockwell, T.K., Meltzner, A.J., Haaker, E.C. and Madugo, D., 2022. The late Holocene history of Lake Cahuilla: Two thousand years of repeated fillings within the Salton Trough, Imperial Valley, California. Quaternary Science Reviews, 282, p.107456. https://doi.org/10.1016/j.quascirev.2022.107456.
Scharer, K.M. and Yule, D., 2020. A maximum rupture model for the southern San Andreas and San Jacinto faults, California, derived from paleoseismic earthquake ages: Observations and limitations. Geophysical Research Letters, 47(15), p.e2020GL088532.https://doi.org/10.1029/2020GL088532.
Sieh, K.E., 1986. Slip rate across the San Andreas fault and prehistoric earthquakes at Indio, California. Eos Trans. AGU, 67(44), p.1200.
Sieh, K.E. and Williams, P.L., 1990. Behavior of the southernmost San Andreas fault during the past 300 years. Journal of Geophysical Research: Solid Earth, 95(B5), pp.6629-6645.https://doi.org/10.1029/JB095iB05p06629.
Thank you for making this and your other blog posts analyzing published papers free to access. It was previously a bit unfair to initiate these discussions (with a very broad audience, including much of the earthquake science community) in a manner such that the original authors could not publicly respond to your analyses without paid subscriptions (I only became aware of this blog post discussing my paper after it was already behind a paywall!) I would still probably encourage submitting comments to the journal for some of your more critical analyses of recent papers, because the comments section of a blog still doesn't really provide the original authors with an adequate medium to respond.
All that said, now that I've finally had the opportunity to read this blog post, I appreciate your evenhanded discussion of the differences between my paper and the recent Hill et al. paper. You do raise an interesting possibility that the soft sediment deformation observed during Coa-1 and Coa-2 may not strictly require a lake highstand. I've had numerous discussions with Tom Rockwell about the reinterpretation of the stratigraphy, and my opinion is that his reinterpretations are plausible, but not incontrovertible, similar to the stance you take in this post. While re-excavation at the Coachella site would be very interesting (we only had ~2.5 weeks-- a crazy small amount of time for the amount of trench there was to interpret), the massive amount of permitting overhead that is required these days makes it unlikely to be worthwhile if the target is mainly hammering out secondary details. Regardless of which interpretation is correct, it wouldn't move the needle a lot in terms of our understanding of earthquake hazards in the region. While the absence of Lake Cahuilla may well have prolonged the current interval between earthquakes, everyone agrees the next earthquake is likely to happen soon, regardless.